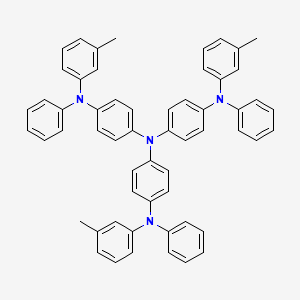
N1-Phenyl-N4,N4-bis(4-(phenyl(m-tolyl)amino)phenyl)-N1-(m-tolyl)benzene-1,4-diamine
- Click on QUICK INQUIRY to receive a quote from our team of experts.
- With the quality product at a COMPETITIVE price, you can focus more on your research.
Overview
Description
“N1-Phenyl-N4,N4-bis(4-(phenyl(m-tolyl)amino)phenyl)-N1-(m-tolyl)benzene-1,4-diamine” is a molecule that has been used as a multifunctional component of exciplex emitters .
Synthesis Analysis
The molecule was synthesized by connecting tercarbazole (3Cz) and triphenyltriazine (TRz) units with a diphenyl ether group .Molecular Structure Analysis
The molecule has a donor-spacer-acceptor structure. The intermolecular charge-transfer transition is dominant for this molecule because of the space-enough and conjugation-forbidden linkage of the diphenyl ether group .Chemical Reactions Analysis
The molecule was used to construct six exciplex emitters with three common electron acceptors and three common electron donors .Physical And Chemical Properties Analysis
The molecule increases the intrinsic characteristics of the 3Cz and TRz moieties in its single-molecule state .Scientific Research Applications
Hole-Injection Buffer Layer (HIL) in OLEDs
m-MTDATA is used as an effective material for the hole-injection buffer layer (HIL) in organic light-emitting diodes (OLEDs). It facilitates hole injection from the indium tin oxide (ITO) electrode to the hole transporting layer (HTL), thanks to its very low solid-state ionization potential and ability to form good-quality amorphous films .
Optoelectronic Devices
Due to its good electron-donor characteristics, m-MTDATA is widely utilized in optoelectronic devices . The starburst π-conjugated molecule based on triphenylamine (TPA) building blocks enhances the electronic structure of these devices .
Organic Ultraviolet Photodetectors
m-MTDATA shows strong photoluminescence quenching when combined with Cu(I) complexes, indicating efficient photoinduced charge transfer. This property is leveraged in organic ultraviolet photodetectors , where m-MTDATA-based devices exhibit high response rates under UV light irradiation .
Mechanism of Action
Target of Action
The primary target of m-MTDATA, also known as N1-Phenyl-N4,N4-bis(4-(phenyl(m-tolyl)amino)phenyl)-N1-(m-tolyl)benzene-1,4-diamine, is the exciplex emitters in organic light-emitting diodes (OLEDs) . These emitters are crucial for the efficient operation of OLEDs.
Mode of Action
m-MTDATA interacts with its targets through an intermolecular charge-transfer transition . This interaction is dominant due to the space-enough and conjugation-forbidden linkage of the diphenyl ether group, which increases the intrinsic characteristics of the 3Cz and TRz moieties in its single-molecule state .
Biochemical Pathways
m-MTDATA affects the biochemical pathways involved in the operation of OLEDs. It is used to construct six exciplex emitters with 3Cz-o-TRz . The compound’s interaction with these emitters modulates the emission spectra of the OLEDs .
Pharmacokinetics
The compound’s structure and mode of action allow it to effectively interact with its targets, thereby influencing the performance of the OLEDs .
Result of Action
The action of m-MTDATA results in the modulation of the emission spectra of OLEDs, with the spectra being gradually adjusted from 510 to 590 nm . Moreover, OLEDs based on TAPC:3Cz-o-TRz and 3Cz-o-TRz:PO-T2T achieved desirable performance with maximum external quantum efficiencies (EQEs) around 12% .
Action Environment
The action, efficacy, and stability of m-MTDATA are influenced by the environment within the OLEDs. For instance, a simple tandem OLED containing TAPC:3Cz-o-TRz and 3Cz-o-TRz:PO-T2T emitters realized optimal performance with an ultralow turn-on voltage of 2.4 V and a maximum EQE of 14.1% .
Future Directions
properties
IUPAC Name |
4-N-(3-methylphenyl)-1-N,1-N-bis[4-(N-(3-methylphenyl)anilino)phenyl]-4-N-phenylbenzene-1,4-diamine |
Source
|
---|---|---|
Source | PubChem | |
URL | https://pubchem.ncbi.nlm.nih.gov | |
Description | Data deposited in or computed by PubChem | |
InChI |
InChI=1S/C57H48N4/c1-43-16-13-25-55(40-43)59(46-19-7-4-8-20-46)52-34-28-49(29-35-52)58(50-30-36-53(37-31-50)60(47-21-9-5-10-22-47)56-26-14-17-44(2)41-56)51-32-38-54(39-33-51)61(48-23-11-6-12-24-48)57-27-15-18-45(3)42-57/h4-42H,1-3H3 |
Source
|
Source | PubChem | |
URL | https://pubchem.ncbi.nlm.nih.gov | |
Description | Data deposited in or computed by PubChem | |
InChI Key |
DIVZFUBWFAOMCW-UHFFFAOYSA-N |
Source
|
Source | PubChem | |
URL | https://pubchem.ncbi.nlm.nih.gov | |
Description | Data deposited in or computed by PubChem | |
Canonical SMILES |
CC1=CC(=CC=C1)N(C2=CC=CC=C2)C3=CC=C(C=C3)N(C4=CC=C(C=C4)N(C5=CC=CC=C5)C6=CC=CC(=C6)C)C7=CC=C(C=C7)N(C8=CC=CC=C8)C9=CC=CC(=C9)C |
Source
|
Source | PubChem | |
URL | https://pubchem.ncbi.nlm.nih.gov | |
Description | Data deposited in or computed by PubChem | |
Molecular Formula |
C57H48N4 |
Source
|
Source | PubChem | |
URL | https://pubchem.ncbi.nlm.nih.gov | |
Description | Data deposited in or computed by PubChem | |
DSSTOX Substance ID |
DTXSID40453692 |
Source
|
Record name | N~1~-(3-Methylphenyl)-N~4~,N~4~-bis{4-[(3-methylphenyl)(phenyl)amino]phenyl}-N~1~-phenylbenzene-1,4-diamine | |
Source | EPA DSSTox | |
URL | https://comptox.epa.gov/dashboard/DTXSID40453692 | |
Description | DSSTox provides a high quality public chemistry resource for supporting improved predictive toxicology. | |
Molecular Weight |
789.0 g/mol |
Source
|
Source | PubChem | |
URL | https://pubchem.ncbi.nlm.nih.gov | |
Description | Data deposited in or computed by PubChem | |
CAS RN |
124729-98-2 |
Source
|
Record name | N~1~-(3-Methylphenyl)-N~4~,N~4~-bis{4-[(3-methylphenyl)(phenyl)amino]phenyl}-N~1~-phenylbenzene-1,4-diamine | |
Source | EPA DSSTox | |
URL | https://comptox.epa.gov/dashboard/DTXSID40453692 | |
Description | DSSTox provides a high quality public chemistry resource for supporting improved predictive toxicology. | |
Disclaimer and Information on In-Vitro Research Products
Please be aware that all articles and product information presented on BenchChem are intended solely for informational purposes. The products available for purchase on BenchChem are specifically designed for in-vitro studies, which are conducted outside of living organisms. In-vitro studies, derived from the Latin term "in glass," involve experiments performed in controlled laboratory settings using cells or tissues. It is important to note that these products are not categorized as medicines or drugs, and they have not received approval from the FDA for the prevention, treatment, or cure of any medical condition, ailment, or disease. We must emphasize that any form of bodily introduction of these products into humans or animals is strictly prohibited by law. It is essential to adhere to these guidelines to ensure compliance with legal and ethical standards in research and experimentation.