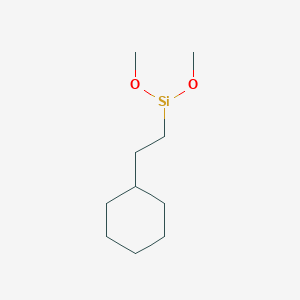
Cyclohexylethyldimethoxysilane
Overview
Description
Cyclohexylethyldimethoxysilane is a chemical compound with the molecular formula C10H22O2Si . It has a molecular weight of 202.37 . It is used as an external donor for Ziegler-Natta catalyst .
Physical and Chemical Properties Analysis
This compound is a colorless liquid . It has a melting point of less than 0°C and a boiling point of 81°C . Its density is 0.938 .Scientific Research Applications
1. Synthesis and Characterization of Multiwall Carbon Nanotubes
Cyclohexanol, related to cyclohexylethyldimethoxysilane, has been employed as a carbon precursor in the synthesis of multiwall carbon nanotubes (MWCNTs) using a Chemical Vapor Deposition (CVD) system. This process highlighted the potential of cyclohexanol in reducing the formation of amorphous carbon and catalyst particles in the as-grown CNTs, indicating its effectiveness in obtaining high purity MWCNTs (Shirazi et al., 2011).
2. Degradation of Recalcitrant Compounds
Studies have shown the effectiveness of cyclohexane, closely related to this compound, in the degradation of recalcitrant compounds. A cyclohexane-degrading consortium was obtained from oil-contaminated soil, highlighting the potential of using such compounds in environmental remediation and pollutant degradation (Lee & Cho, 2008).
3. Liquid-Phase Catalytic Oxidation
Cyclohexane's transformation into adipic acid via cyclohexanol–cyclohexanone mixture formation has been analyzed in industrial processes. This highlights the role of cyclohexane-related compounds in catalytic oxidation, an essential process in chemical industry applications (Brégeault, 2003).
4. Enantioselective Oxidation in Organic Synthesis
Cyclohexanone monooxygenases, related to cyclohexane compounds, have been applied for the enantioselective oxidation of organic sulfur compounds to sulfoxides. This application is significant in organic synthesis, demonstrating the versatility of cyclohexane derivatives in catalyzing important chemical reactions (Colonna et al., 1996).
5. Polymerization and Catalysis
Research indicates the utility of cyclohexene, a compound structurally related to this compound, in ethylene/cyclohexene copolymerization. This process, catalyzed by nonbridged half-titanocenes, emphasizes the role of cyclohexane derivatives in polymer science and catalysis (Wang et al., 2005).
Safety and Hazards
Cyclohexylethyldimethoxysilane is classified as a skin irritant (Category 2) and is hazardous to the aquatic environment with long-lasting effects (Chronic Category 2) . Safety measures include wearing protective gear and avoiding release to the environment . In case of skin contact, it is advised to wash with plenty of water and seek medical help if skin irritation occurs .
Properties
IUPAC Name |
cyclohexyl-ethyl-dimethoxysilane | |
---|---|---|
Source | PubChem | |
URL | https://pubchem.ncbi.nlm.nih.gov | |
Description | Data deposited in or computed by PubChem | |
InChI |
InChI=1S/C10H22O2Si/c1-4-13(11-2,12-3)10-8-6-5-7-9-10/h10H,4-9H2,1-3H3 | |
Source | PubChem | |
URL | https://pubchem.ncbi.nlm.nih.gov | |
Description | Data deposited in or computed by PubChem | |
InChI Key |
QEPVYYOIYSITJK-UHFFFAOYSA-N | |
Source | PubChem | |
URL | https://pubchem.ncbi.nlm.nih.gov | |
Description | Data deposited in or computed by PubChem | |
Canonical SMILES |
CC[Si](C1CCCCC1)(OC)OC | |
Source | PubChem | |
URL | https://pubchem.ncbi.nlm.nih.gov | |
Description | Data deposited in or computed by PubChem | |
Molecular Formula |
C10H22O2Si | |
Source | PubChem | |
URL | https://pubchem.ncbi.nlm.nih.gov | |
Description | Data deposited in or computed by PubChem | |
Molecular Weight |
202.37 g/mol | |
Source | PubChem | |
URL | https://pubchem.ncbi.nlm.nih.gov | |
Description | Data deposited in or computed by PubChem | |
CAS No. |
131390-30-2 | |
Record name | Cyclohexylethyldimethoxysilane | |
Source | CAS Common Chemistry | |
URL | https://commonchemistry.cas.org/detail?cas_rn=131390-30-2 | |
Description | CAS Common Chemistry is an open community resource for accessing chemical information. Nearly 500,000 chemical substances from CAS REGISTRY cover areas of community interest, including common and frequently regulated chemicals, and those relevant to high school and undergraduate chemistry classes. This chemical information, curated by our expert scientists, is provided in alignment with our mission as a division of the American Chemical Society. | |
Explanation | The data from CAS Common Chemistry is provided under a CC-BY-NC 4.0 license, unless otherwise stated. | |
Retrosynthesis Analysis
AI-Powered Synthesis Planning: Our tool employs the Template_relevance Pistachio, Template_relevance Bkms_metabolic, Template_relevance Pistachio_ringbreaker, Template_relevance Reaxys, Template_relevance Reaxys_biocatalysis model, leveraging a vast database of chemical reactions to predict feasible synthetic routes.
One-Step Synthesis Focus: Specifically designed for one-step synthesis, it provides concise and direct routes for your target compounds, streamlining the synthesis process.
Accurate Predictions: Utilizing the extensive PISTACHIO, BKMS_METABOLIC, PISTACHIO_RINGBREAKER, REAXYS, REAXYS_BIOCATALYSIS database, our tool offers high-accuracy predictions, reflecting the latest in chemical research and data.
Strategy Settings
Precursor scoring | Relevance Heuristic |
---|---|
Min. plausibility | 0.01 |
Model | Template_relevance |
Template Set | Pistachio/Bkms_metabolic/Pistachio_ringbreaker/Reaxys/Reaxys_biocatalysis |
Top-N result to add to graph | 6 |
Feasible Synthetic Routes
Disclaimer and Information on In-Vitro Research Products
Please be aware that all articles and product information presented on BenchChem are intended solely for informational purposes. The products available for purchase on BenchChem are specifically designed for in-vitro studies, which are conducted outside of living organisms. In-vitro studies, derived from the Latin term "in glass," involve experiments performed in controlled laboratory settings using cells or tissues. It is important to note that these products are not categorized as medicines or drugs, and they have not received approval from the FDA for the prevention, treatment, or cure of any medical condition, ailment, or disease. We must emphasize that any form of bodily introduction of these products into humans or animals is strictly prohibited by law. It is essential to adhere to these guidelines to ensure compliance with legal and ethical standards in research and experimentation.