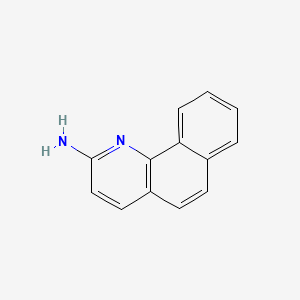
Benzo(h)quinoline, 2-amino-
- Click on QUICK INQUIRY to receive a quote from our team of experts.
- With the quality product at a COMPETITIVE price, you can focus more on your research.
Overview
Description
Benzo[h]quinolin-2-amine is a nitrogen-containing heterocyclic compound, part of the quinoline family. This compound is characterized by a fused benzene and pyridine ring system, with an amine group attached at the second position. Its unique structure imparts significant chemical reactivity and potential for various applications in medicinal and industrial chemistry.
Preparation Methods
Synthetic Routes and Reaction Conditions: Benzo[h]quinolin-2-amine can be synthesized through several methods. One common approach involves the reaction of naphthalen-1-amine with appropriate precursors under specific conditions. For instance, the reaction of mercaptoacetic acid esters with pentachloro-2-nitro-1,3-butadiene provides the necessary intermediates for the synthesis of benzo[h]quinolin-2-amine . This reaction typically occurs under solvent-free conditions and may involve microwave irradiation to enhance the reaction rate .
Industrial Production Methods: Industrial production of benzo[h]quinolin-2-amine often employs green chemistry principles to minimize environmental impact. Methods such as one-pot reactions, solvent-free conditions, and the use of recyclable catalysts are preferred . These approaches not only improve the efficiency of the synthesis but also align with sustainable chemical practices.
Chemical Reactions Analysis
Types of Reactions: Benzo[h]quinolin-2-amine undergoes various chemical reactions, including:
Oxidation: This compound can be oxidized to form quinoline N-oxides.
Reduction: Reduction reactions can convert benzo[h]quinolin-2-amine to its corresponding amine derivatives.
Substitution: Nucleophilic and electrophilic substitution reactions are common, given the presence of the amine group and the aromatic ring system.
Common Reagents and Conditions:
Oxidation: Reagents such as hydrogen peroxide or peracids are commonly used.
Reduction: Catalytic hydrogenation or the use of reducing agents like lithium aluminum hydride.
Substitution: Halogenating agents, alkylating agents, and various nucleophiles can be employed under appropriate conditions.
Major Products: The major products formed from these reactions include various substituted quinoline derivatives, which can exhibit significant biological activity .
Scientific Research Applications
Benzo[h]quinolin-2-amine has a wide range of applications in scientific research:
Chemistry: It serves as a building block for the synthesis of more complex heterocyclic compounds.
Biology: Its derivatives are studied for their potential as enzyme inhibitors and receptor modulators.
Industry: It is used in the synthesis of dyes, pigments, and other industrial chemicals.
Mechanism of Action
The mechanism of action of benzo[h]quinolin-2-amine involves its interaction with various molecular targets. For instance, its derivatives can inhibit enzymes by binding to their active sites, thereby blocking substrate access. Additionally, it can modulate receptor activity by interacting with specific binding sites on the receptor molecules . These interactions often involve hydrogen bonding, π-π stacking, and other non-covalent interactions.
Comparison with Similar Compounds
Quinoline: A simpler analog with a similar fused ring system but lacking the amine group.
Isoquinoline: Another isomeric form with the nitrogen atom in a different position.
4-Hydroxy-2-quinolones: Compounds with hydroxyl and carbonyl groups that exhibit different reactivity and biological activity.
Uniqueness: Benzo[h]quinolin-2-amine is unique due to its specific substitution pattern, which imparts distinct chemical reactivity and biological activity. Its ability to undergo a wide range of chemical reactions and its potential for diverse applications make it a valuable compound in both research and industry .
Biological Activity
Benzo(h)quinoline, particularly its 2-amino derivative, has garnered attention in recent years due to its diverse biological activities, particularly in the field of oncology. This article explores the synthesis, biological evaluation, and mechanisms of action related to 2-amino-benzo(h)quinoline, with a focus on its anticancer properties.
Synthesis of 2-Amino-Benzo(h)quinoline Derivatives
The synthesis of 2-amino-benzo(h)quinoline derivatives typically involves multi-step reactions. A common method includes the condensation of arylidene-1-tetralone with malononitrile under various conditions, such as microwave irradiation or conventional heating. This approach has been shown to yield compounds with high purity and efficiency .
Table 1: Common Synthesis Methods for 2-Amino-Benzo(h)quinoline
Anticancer Activity
Numerous studies have demonstrated the anticancer potential of 2-amino-benzo(h)quinoline derivatives against various cancer cell lines. The mechanisms of action include:
- Induction of Apoptosis : Compounds have been shown to increase intracellular reactive oxygen species (ROS), leading to DNA damage and apoptosis in cancer cells. For instance, a study reported that specific derivatives exhibited IC50 values ranging from 4.7 to 7.6 µM against human cancer cell lines including G361 (skin), H460 (lung), MCF7 (breast), and HCT116 (colon) .
- Inhibition of Cyclin-dependent Kinases : The compounds target cyclin-dependent kinase-2 (CDK2), a crucial regulator of cell cycle progression. Inhibition of CDK2 leads to cell cycle arrest and subsequent apoptosis .
- DNA Intercalation : Some derivatives can intercalate into the DNA double helix, disrupting normal cellular functions and promoting cytotoxicity .
Table 2: Anticancer Activity of Selected 2-Amino-Benzo(h)quinoline Derivatives
Compound | Cancer Cell Line | IC50 (µM) | Mechanism of Action |
---|---|---|---|
Compound 3e | G361 | 4.7 | CDK2 inhibition |
Compound 3f | H460 | 5.3 | Induction of apoptosis |
Compound 3h | MCF7 | 6.0 | DNA intercalation |
Compound 3j | HCT116 | 7.6 | ROS generation |
Case Studies
- Study on Glioblastoma Cells : A study evaluated the cytotoxic effects of various benzo(h)quinoline derivatives on U373 glioblastoma cells using the WST-1 assay. Results indicated that certain derivatives caused significant cell death (up to 80% at optimal concentrations), highlighting their potential as therapeutic agents against aggressive brain tumors .
- Mechanistic Insights : Research has shown that treatment with benzo(h)quinoline derivatives led to the overexpression of oxidative stress-related genes such as ATM and H2AX in cancer cells, which are pivotal in the DNA damage response pathway. This suggests that these compounds not only induce direct cytotoxic effects but also activate cellular repair mechanisms that can lead to apoptosis when overwhelmed .
Properties
CAS No. |
67410-22-4 |
---|---|
Molecular Formula |
C13H10N2 |
Molecular Weight |
194.23 g/mol |
IUPAC Name |
benzo[h]quinolin-2-amine |
InChI |
InChI=1S/C13H10N2/c14-12-8-7-10-6-5-9-3-1-2-4-11(9)13(10)15-12/h1-8H,(H2,14,15) |
InChI Key |
ADDJIAQBVKRVFQ-UHFFFAOYSA-N |
Canonical SMILES |
C1=CC=C2C(=C1)C=CC3=C2N=C(C=C3)N |
Origin of Product |
United States |
Disclaimer and Information on In-Vitro Research Products
Please be aware that all articles and product information presented on BenchChem are intended solely for informational purposes. The products available for purchase on BenchChem are specifically designed for in-vitro studies, which are conducted outside of living organisms. In-vitro studies, derived from the Latin term "in glass," involve experiments performed in controlled laboratory settings using cells or tissues. It is important to note that these products are not categorized as medicines or drugs, and they have not received approval from the FDA for the prevention, treatment, or cure of any medical condition, ailment, or disease. We must emphasize that any form of bodily introduction of these products into humans or animals is strictly prohibited by law. It is essential to adhere to these guidelines to ensure compliance with legal and ethical standards in research and experimentation.