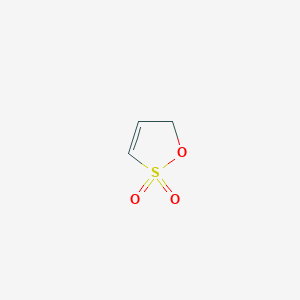
Prop-1-ene-1,3-sultone
Overview
Description
Prop-1-ene-1,3-sultone: is a chemical compound with the molecular formula C3H4O3S. It is characterized by the presence of a sultone functional group, which is a cyclic sulfonate ester. This compound is known for its applications in various chemical processes, including organic synthesis, polymer production, and electrochemical devices .
Mechanism of Action
Target of Action
Prop-1-ene-1,3-sultone (PES) is primarily used as an electrolyte additive in lithium-ion batteries . Its primary target is the solid electrolyte interphase (SEI) film on the anode of the battery . The SEI film plays a crucial role in the performance of the battery by minimizing the loss of lithium and decomposition of the electrolyte .
Mode of Action
PES interacts with its target, the SEI film, through a process of reductive decomposition . This process involves three ring-opening pathways: O–C, S–C, and S–O bond-breaking processes . The Li+ ion plays a pivotal role in the reductive decomposition of PES . While the most kinetically favored process—the S–O bond breaking—is effectively blocked via the formation of an intermediate structure, namely, the Li±participated seven-membered ring, the other decomposition processes via O–C and S–C bond breaking lead to stable decomposition products .
Biochemical Pathways
The decomposition of PES leads to the formation of sulfates and sulfites (such as ROSO2Na and RSO3Na) through the O–C and S–C bond-breaking processes . These products can stabilize the SEI film, contributing to the high performance and long lifespan of the battery .
Pharmacokinetics
While the term “pharmacokinetics” is typically used in the context of drug metabolism, in the case of PES, we can discuss its behavior in the battery environment. The reduction of PES occurs via two one-electron steps . The reduced species, Li2PES, can react with hydrogen and methyl radicals to produce propene, methylpropene, propane, and lithium sulfite .
Result of Action
The result of PES’s action is the formation of a more stable and effective SEI film on the anode of the lithium-ion battery . This leads to remarkable cell performance in lithium-ion batteries . The constituents of SEI observed in previous experimental studies, such as RSO3Li and ROSO2Li, can be reasonably understood as the decomposition products resulting from O–C and S–C bond breaking, respectively .
Action Environment
The action of PES is influenced by the battery environment. For instance, adding PES in the base electrolyte can achieve an increasingly capacity retention rate . Pes has a negative effect when added in certain proportions . Electron microscopy characterizations and X-ray photoelectron spectroscopy results confirm that PES can generate a denser and more uniform SEI film on the electrode, meanwhile, be oxidized on the positive electrode to protect the material from the attack of the electrolyte during cycling .
Biochemical Analysis
Biochemical Properties
Prop-1-ene-1,3-sultone plays a significant role in biochemical reactions, particularly as an additive in electrolyte solutions for batteries. It interacts with various enzymes, proteins, and other biomolecules to form a stable solid electrolyte interphase (SEI) film. This interaction is crucial for improving the cyclability and performance of batteries. The compound’s ability to form a dense and uniform SEI film is attributed to its interaction with sulfates and sulfites, such as ROSO2Na and RSO3Na, which stabilize the SEI film .
Cellular Effects
This compound influences various types of cells and cellular processes. It has been observed to affect cell signaling pathways, gene expression, and cellular metabolism. The compound’s interaction with cellular components leads to the formation of a protective layer that prevents degradation and enhances cell function. This protective layer is particularly important in maintaining the stability and performance of cells in battery applications .
Molecular Mechanism
The molecular mechanism of this compound involves its binding interactions with biomolecules, enzyme inhibition or activation, and changes in gene expression. The compound undergoes oxidation at the positive electrode, producing carbonyl sulfide gas and ethene as byproducts. These byproducts are experimentally observed and contribute to the compound’s overall effect on the system. The formation of a stable SEI film is a key aspect of its molecular mechanism .
Temporal Effects in Laboratory Settings
In laboratory settings, the effects of this compound change over time. The compound’s stability and degradation are critical factors that influence its long-term effects on cellular function. Studies have shown that the compound can maintain its effectiveness over extended periods, contributing to the longevity and performance of cells in battery applications. The degradation of the compound can lead to a decrease in its protective properties .
Dosage Effects in Animal Models
The effects of this compound vary with different dosages in animal models. At lower dosages, the compound exhibits beneficial effects, such as improved cell function and stability. At higher dosages, toxic or adverse effects may be observed. These threshold effects are important for determining the optimal dosage for various applications .
Metabolic Pathways
This compound is involved in several metabolic pathways, interacting with enzymes and cofactors to influence metabolic flux and metabolite levels. The compound’s role in forming a stable SEI film is linked to its interaction with sulfates and sulfites, which are key components of its metabolic pathways. These interactions contribute to the compound’s overall effectiveness in various applications .
Transport and Distribution
The transport and distribution of this compound within cells and tissues are facilitated by specific transporters and binding proteins. These interactions influence the compound’s localization and accumulation, which are critical for its function. The compound’s ability to form a protective layer is dependent on its distribution within the cellular environment .
Subcellular Localization
This compound is localized within specific subcellular compartments, where it exerts its activity and function. Targeting signals and post-translational modifications direct the compound to these compartments, ensuring its effectiveness. The compound’s subcellular localization is crucial for its role in forming a stable SEI film and enhancing cell function .
Preparation Methods
Synthetic Routes and Reaction Conditions:
Halogenation Method: Prop-1-ene-1,3-sultone can be synthesized by halogenating this compound to obtain a 3-halogenated intermediate.
Chlorination and Bromination Method: Another method involves chlorinating POCl3 or PCl5 with a specific compound to produce an intermediate, which is then brominated in dichloroethylene solvent.
Industrial Production Methods: Industrial production of this compound typically involves large-scale synthesis using the above methods, ensuring high purity and yield. The reaction conditions are optimized to achieve efficient production with minimal byproducts .
Chemical Reactions Analysis
Types of Reactions:
Reduction: The compound can be reduced to form different reduced species, which can further react with other compounds.
Substitution: It can participate in substitution reactions, where the sultone group is replaced by other functional groups.
Common Reagents and Conditions:
Oxidation: Common oxidizing agents include hydrogen peroxide and singlet oxygen.
Reduction: Reducing agents such as lithium ions are used in the reduction process.
Substitution: Various nucleophiles can be used for substitution reactions under appropriate conditions.
Major Products Formed:
Oxidation Products: Singlet oxygen and hydrogen peroxide.
Reduction Products: Stable decomposition products such as RSO3Li and ROSO2Li.
Substitution Products: Various substituted sultone derivatives.
Scientific Research Applications
Chemistry: Prop-1-ene-1,3-sultone is used as a reagent in organic synthesis, providing a versatile platform for introducing the sultone moiety into different molecules .
Biology: The compound’s ability to generate singlet oxygen and hydrogen peroxide makes it useful in biological studies involving oxidative stress and related processes .
Industry: this compound is used as an additive in electrolytes for lithium-ion batteries, enhancing the stability and performance of the batteries .
Comparison with Similar Compounds
1,3-Propane Sultone: Similar in structure but differs in its applications and reactivity.
Ethylene Sulfate: Another sulfur-based additive used in battery electrolytes.
1,4-Butane Sultone: Used as an electrolyte additive with different properties compared to Prop-1-ene-1,3-sultone.
Uniqueness: this compound is unique due to its ability to form effective SEI films in lithium-ion batteries, enhancing their stability and performance. Its specific reactivity and applications in various fields make it distinct from other similar compounds .
Properties
IUPAC Name |
5H-oxathiole 2,2-dioxide | |
---|---|---|
Source | PubChem | |
URL | https://pubchem.ncbi.nlm.nih.gov | |
Description | Data deposited in or computed by PubChem | |
InChI |
InChI=1S/C3H4O3S/c4-7(5)3-1-2-6-7/h1,3H,2H2 | |
Source | PubChem | |
URL | https://pubchem.ncbi.nlm.nih.gov | |
Description | Data deposited in or computed by PubChem | |
InChI Key |
KLLQVNFCMHPYGL-UHFFFAOYSA-N | |
Source | PubChem | |
URL | https://pubchem.ncbi.nlm.nih.gov | |
Description | Data deposited in or computed by PubChem | |
Canonical SMILES |
C1C=CS(=O)(=O)O1 | |
Source | PubChem | |
URL | https://pubchem.ncbi.nlm.nih.gov | |
Description | Data deposited in or computed by PubChem | |
Molecular Formula |
C3H4O3S | |
Source | PubChem | |
URL | https://pubchem.ncbi.nlm.nih.gov | |
Description | Data deposited in or computed by PubChem | |
DSSTOX Substance ID |
DTXSID10447528 | |
Record name | Prop-1-ene-1,3-sultone | |
Source | EPA DSSTox | |
URL | https://comptox.epa.gov/dashboard/DTXSID10447528 | |
Description | DSSTox provides a high quality public chemistry resource for supporting improved predictive toxicology. | |
Molecular Weight |
120.13 g/mol | |
Source | PubChem | |
URL | https://pubchem.ncbi.nlm.nih.gov | |
Description | Data deposited in or computed by PubChem | |
CAS No. |
21806-61-1 | |
Record name | 5H-1,2-Oxathiole, 2,2-dioxide | |
Source | CAS Common Chemistry | |
URL | https://commonchemistry.cas.org/detail?cas_rn=21806-61-1 | |
Description | CAS Common Chemistry is an open community resource for accessing chemical information. Nearly 500,000 chemical substances from CAS REGISTRY cover areas of community interest, including common and frequently regulated chemicals, and those relevant to high school and undergraduate chemistry classes. This chemical information, curated by our expert scientists, is provided in alignment with our mission as a division of the American Chemical Society. | |
Explanation | The data from CAS Common Chemistry is provided under a CC-BY-NC 4.0 license, unless otherwise stated. | |
Record name | Prop-1-ene-1,3-sultone | |
Source | EPA DSSTox | |
URL | https://comptox.epa.gov/dashboard/DTXSID10447528 | |
Description | DSSTox provides a high quality public chemistry resource for supporting improved predictive toxicology. | |
Record name | 5H-1,2-oxathiole 2,2-dioxide | |
Source | European Chemicals Agency (ECHA) | |
URL | https://echa.europa.eu/substance-information/-/substanceinfo/100.125.830 | |
Description | The European Chemicals Agency (ECHA) is an agency of the European Union which is the driving force among regulatory authorities in implementing the EU's groundbreaking chemicals legislation for the benefit of human health and the environment as well as for innovation and competitiveness. | |
Explanation | Use of the information, documents and data from the ECHA website is subject to the terms and conditions of this Legal Notice, and subject to other binding limitations provided for under applicable law, the information, documents and data made available on the ECHA website may be reproduced, distributed and/or used, totally or in part, for non-commercial purposes provided that ECHA is acknowledged as the source: "Source: European Chemicals Agency, http://echa.europa.eu/". Such acknowledgement must be included in each copy of the material. ECHA permits and encourages organisations and individuals to create links to the ECHA website under the following cumulative conditions: Links can only be made to webpages that provide a link to the Legal Notice page. | |
Retrosynthesis Analysis
AI-Powered Synthesis Planning: Our tool employs the Template_relevance Pistachio, Template_relevance Bkms_metabolic, Template_relevance Pistachio_ringbreaker, Template_relevance Reaxys, Template_relevance Reaxys_biocatalysis model, leveraging a vast database of chemical reactions to predict feasible synthetic routes.
One-Step Synthesis Focus: Specifically designed for one-step synthesis, it provides concise and direct routes for your target compounds, streamlining the synthesis process.
Accurate Predictions: Utilizing the extensive PISTACHIO, BKMS_METABOLIC, PISTACHIO_RINGBREAKER, REAXYS, REAXYS_BIOCATALYSIS database, our tool offers high-accuracy predictions, reflecting the latest in chemical research and data.
Strategy Settings
Precursor scoring | Relevance Heuristic |
---|---|
Min. plausibility | 0.01 |
Model | Template_relevance |
Template Set | Pistachio/Bkms_metabolic/Pistachio_ringbreaker/Reaxys/Reaxys_biocatalysis |
Top-N result to add to graph | 6 |
Feasible Synthetic Routes
Q1: How does PES enhance the performance of lithium-ion batteries?
A1: PES acts as a solid-electrolyte interphase (SEI) forming additive in lithium-ion batteries. [, , ] It forms a stable SEI layer on both the anode and cathode, improving battery performance by:
- Reducing gas evolution: PES effectively suppresses gas formation during battery operation, particularly at elevated temperatures. [, , , , ]
- Enhancing coulombic efficiency: Cells with PES exhibit higher coulombic efficiency compared to those without additives or with other additives like vinylene carbonate (VC). [, , ]
- Improving capacity retention: PES-containing cells demonstrate improved capacity retention, especially at high temperatures and high voltages. [, , , ]
- Lowering impedance growth: While PES initially increases charge transfer resistance, it effectively reduces impedance growth during long-term cycling, particularly at high voltages. [, ]
Q2: How does the concentration of PES in the electrolyte affect battery performance?
A2: Increasing PES concentration generally leads to:
- Reduced gas evolution: Higher concentrations further suppress gas formation. []
- Increased charge transfer resistance: This effect is particularly noticeable after formation. []
- Improved capacity retention: Higher concentrations, up to a certain point, can lead to better capacity retention during cycling. []
Q3: Does PES improve battery performance at high temperatures?
A3: Yes, PES has been shown to significantly enhance the performance of lithium-ion batteries at elevated temperatures (e.g., 60°C). [, , ] It forms a more stable SEI layer that can withstand the challenges posed by high temperatures, resulting in reduced capacity fade and improved overall stability.
Q4: What happens when PES is combined with other electrolyte additives?
A4: Combining PES with other electrolyte additives can lead to synergistic effects, further improving battery performance. [, , ] For instance, ternary blends of PES with a sulfur-containing molecule like methylene methanedisulfonate (MMDS) and tris(trimethylsilyl) phosphite (TTSPi) have demonstrated exceptional capacity retention, low impedance, and minimal gas evolution. [, ]
Q5: How does PES interact with the electrodes in a lithium-ion battery?
A5: PES preferentially decomposes at the electrode surfaces during the initial charging cycles of a lithium-ion battery. This decomposition forms a stable SEI layer on both the anode and cathode. [, , , ] The SEI layer acts as a protective barrier, preventing further unwanted reactions between the electrode materials and the electrolyte.
Q6: What are the downstream effects of PES decomposition on the battery?
A6: The decomposition of PES and the subsequent formation of the SEI layer have several beneficial downstream effects:
- Reduced electrolyte decomposition: The SEI layer prevents direct contact between the electrolyte and the electrode surfaces, minimizing further electrolyte decomposition. []
- Suppressed gas formation: By forming a stable SEI, PES reduces parasitic reactions that lead to gas evolution, particularly at elevated temperatures. [, , ]
- Improved lithium-ion diffusion: The SEI layer formed by PES decomposition can facilitate lithium-ion transport, leading to enhanced rate capability and power output. [, ]
- Enhanced cycle life: The stable SEI layer protects the electrode materials from degradation, contributing to a longer cycle life for the battery. [, , ]
Q7: How does the structure of the SEI formed by PES impact its effectiveness?
A7: The SEI formed by PES is believed to be a complex mixture of organic and inorganic compounds, including lithium salts of the decomposed additive. [, , ] The specific composition and morphology of the SEI can influence its effectiveness in protecting the electrodes and facilitating lithium-ion transport. Research suggests that a thicker and denser SEI layer with low resistance to Li-ion conduction contributes to better battery performance. []
Q8: How is computational chemistry used to study the behavior of PES in batteries?
A8: Density functional theory (DFT) calculations are employed to investigate the decomposition mechanisms of PES in lithium-ion battery electrolytes. [, , ] These calculations provide insights into:
- Reduction potentials: DFT can predict the reduction potential of PES, which is crucial for understanding its decomposition behavior during battery operation. [, ]
- Decomposition pathways: By simulating the interactions between PES and other electrolyte components, researchers can identify the most likely decomposition products and pathways. [, ]
- SEI composition: DFT calculations can help predict the composition of the SEI layer formed by PES decomposition, enabling researchers to correlate SEI properties with battery performance. [, ]
Q9: Can computational methods be used to design better electrolyte additives?
A9: Yes, computational chemistry plays a crucial role in guiding the development of novel electrolyte additives with improved performance. [] By using DFT calculations and other modeling techniques, researchers can:
Q10: What is the molecular formula and weight of prop-1-ene-1,3-sultone?
A10: The molecular formula of this compound (PES) is C3H4O3S, and its molecular weight is 120.13 g/mol.
Q11: What spectroscopic techniques are used to characterize PES?
A11: Common spectroscopic techniques used to characterize PES and the SEI layer it forms include:
- X-ray photoelectron spectroscopy (XPS): XPS provides information about the elemental composition and chemical states of the SEI layer formed on the electrode surfaces. [, , , , ]
- Gas chromatography-mass spectrometry (GC-MS): GC-MS is used to identify and quantify the gaseous products formed during battery operation, providing insights into the decomposition pathways of PES and the electrolyte. [, ]
Q12: Are there any safety concerns associated with the use of PES?
A12: As with any chemical used in battery systems, safety considerations are paramount. While PES has shown promise in improving battery performance, it's essential to consider potential hazards:
Q13: What are the key areas of ongoing research related to PES?
A13: Ongoing research focuses on several key aspects of PES:
Q14: What are the potential applications of PES beyond lithium-ion batteries?
A14: While the primary focus of PES research is on lithium-ion batteries, its SEI-forming properties could potentially be beneficial in other battery chemistries:
Disclaimer and Information on In-Vitro Research Products
Please be aware that all articles and product information presented on BenchChem are intended solely for informational purposes. The products available for purchase on BenchChem are specifically designed for in-vitro studies, which are conducted outside of living organisms. In-vitro studies, derived from the Latin term "in glass," involve experiments performed in controlled laboratory settings using cells or tissues. It is important to note that these products are not categorized as medicines or drugs, and they have not received approval from the FDA for the prevention, treatment, or cure of any medical condition, ailment, or disease. We must emphasize that any form of bodily introduction of these products into humans or animals is strictly prohibited by law. It is essential to adhere to these guidelines to ensure compliance with legal and ethical standards in research and experimentation.