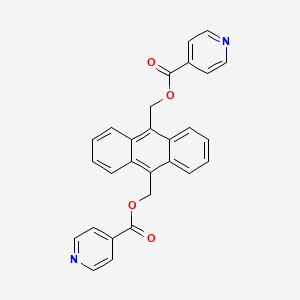
Anthracene-9,10-diylbis(methylene) diisonicotinate
- Click on QUICK INQUIRY to receive a quote from our team of experts.
- With the quality product at a COMPETITIVE price, you can focus more on your research.
Overview
Description
Anthracene-9,10-diylbis(methylene) diisonicotinate is a compound derived from anthracene, a polycyclic aromatic hydrocarbon. This compound is known for its unique structural properties, which make it useful in various scientific and industrial applications. The compound consists of an anthracene core with two methylene bridges connecting to isonicotinate groups.
Preparation Methods
Synthetic Routes and Reaction Conditions
The synthesis of Anthracene-9,10-diylbis(methylene) diisonicotinate typically involves the reaction of anthracene-9,10-diylbis(methylene) with isonicotinic acid. The reaction is carried out in the presence of a suitable catalyst and under controlled temperature and pressure conditions to ensure high yield and purity.
Industrial Production Methods
In industrial settings, the production of this compound may involve large-scale reactions using continuous flow reactors. These reactors allow for precise control over reaction conditions, leading to consistent product quality. The use of advanced purification techniques, such as chromatography, ensures that the final product meets the required specifications.
Chemical Reactions Analysis
Types of Reactions
Anthracene-9,10-diylbis(methylene) diisonicotinate undergoes various chemical reactions, including:
Oxidation: The compound can be oxidized to form corresponding quinones.
Reduction: Reduction reactions can convert the compound into its hydro derivatives.
Substitution: The isonicotinate groups can be substituted with other functional groups under appropriate conditions.
Common Reagents and Conditions
Oxidation: Common oxidizing agents include potassium permanganate and chromium trioxide.
Reduction: Reducing agents such as sodium borohydride and lithium aluminum hydride are used.
Substitution: Substitution reactions often require catalysts like palladium on carbon and specific solvents to facilitate the reaction.
Major Products Formed
Oxidation: Anthracene-9,10-dione derivatives.
Reduction: Hydro-anthracene derivatives.
Substitution: Various substituted anthracene derivatives depending on the substituent introduced.
Scientific Research Applications
Anthracene-9,10-diylbis(methylene) diisonicotinate has a wide range of applications in scientific research:
Mechanism of Action
The mechanism of action of Anthracene-9,10-diylbis(methylene) diisonicotinate involves its interaction with molecular targets such as lipopolysaccharides in cell membranes. The compound’s fluorescent properties enable it to act as a probe, emitting light upon excitation. This emission can be used to monitor various biological and chemical processes. Additionally, its ability to generate singlet oxygen makes it useful in photodynamic therapy, where it induces oxidative stress in target cells .
Comparison with Similar Compounds
Similar Compounds
9,10-Anthracenediyl-bis(methylene)dimalonic acid: Another derivative of anthracene used as a singlet oxygen probe.
Anthracene-9,10-diylbis(methylene) diisonicotinate: Known for its unique structural properties and applications in MOFs.
Uniqueness
This compound stands out due to its dual functionality as both a fluorescent probe and a component in MOFs. Its ability to selectively bind to specific molecules and generate singlet oxygen makes it a versatile tool in various scientific fields.
Properties
Molecular Formula |
C28H20N2O4 |
---|---|
Molecular Weight |
448.5 g/mol |
IUPAC Name |
[10-(pyridine-4-carbonyloxymethyl)anthracen-9-yl]methyl pyridine-4-carboxylate |
InChI |
InChI=1S/C28H20N2O4/c31-27(19-9-13-29-14-10-19)33-17-25-21-5-1-2-6-22(21)26(24-8-4-3-7-23(24)25)18-34-28(32)20-11-15-30-16-12-20/h1-16H,17-18H2 |
InChI Key |
BPGOXOIHKUIEBM-UHFFFAOYSA-N |
Canonical SMILES |
C1=CC=C2C(=C1)C(=C3C=CC=CC3=C2COC(=O)C4=CC=NC=C4)COC(=O)C5=CC=NC=C5 |
Origin of Product |
United States |
Disclaimer and Information on In-Vitro Research Products
Please be aware that all articles and product information presented on BenchChem are intended solely for informational purposes. The products available for purchase on BenchChem are specifically designed for in-vitro studies, which are conducted outside of living organisms. In-vitro studies, derived from the Latin term "in glass," involve experiments performed in controlled laboratory settings using cells or tissues. It is important to note that these products are not categorized as medicines or drugs, and they have not received approval from the FDA for the prevention, treatment, or cure of any medical condition, ailment, or disease. We must emphasize that any form of bodily introduction of these products into humans or animals is strictly prohibited by law. It is essential to adhere to these guidelines to ensure compliance with legal and ethical standards in research and experimentation.