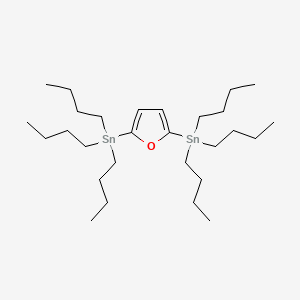
2,5-Bis(tri-n-butylstannyl)furan
Overview
Description
2,5-Bis(tri-n-butylstannyl)furan is an organic compound with the molecular formula C28H56OSn2 and a molecular weight of 646.17 g/mol . It consists of a furan ring with tri-n-butylstannyl groups attached to the 2nd and 5th positions of the ring . This compound is primarily used in synthetic organic chemistry as a reagent and intermediate in various chemical reactions .
Preparation Methods
2,5-Bis(tri-n-butylstannyl)furan can be synthesized through several methods. One common synthetic route involves the reaction of furan with tri-n-butylstannyl chloride in the presence of a base such as sodium hydride . The reaction typically takes place under an inert atmosphere, such as nitrogen or argon, to prevent oxidation. The product is then purified through column chromatography or recrystallization .
Chemical Reactions Analysis
2,5-Bis(tri-n-butylstannyl)furan undergoes various chemical reactions, including:
Substitution Reactions: It can react with halogens such as bromine to form 2,5-bis(tri-n-butyltin)dibromofuran.
Coupling Reactions: It is a common reagent in Suzuki coupling reactions with diamidines and dicyano compounds.
Hydrolysis Reactions: In the presence of water, it can undergo hydrolysis to replace a butyl group with a hydroxyl group.
Common reagents used in these reactions include halides, amines, and methyl iodide . Major products formed from these reactions include dibromofuran and various substituted furans .
Scientific Research Applications
Synthetic Organic Chemistry
Regiospecific Synthesis of Polysubstituted Furans:
2,5-Bis(tri-n-butylstannyl)furan is pivotal in the regiospecific synthesis of polysubstituted furans, which are essential structures in many natural products with biological activities. It participates in Stille coupling reactions, allowing the formation of new carbon-carbon bonds between stannanes and various organic halides or pseudohalides. This capability enables the introduction of furan rings into diverse chemical structures.
Case Study:
A study demonstrated that using this compound in palladium-catalyzed reactions with aryl bromides yielded 2,5-diarylfurans in good yields (up to 86%) . This method provides a convenient one-step process for preparing complex furan derivatives that are often difficult to obtain through traditional methods.
Agrochemical Research
Intermediate for Agrochemical Development:
The compound serves as an intermediate in synthesizing new agrochemicals with desired properties. Its role in developing environmentally friendly pesticides and herbicides is being explored.
Research Findings:
Research indicates that derivatives synthesized from this compound exhibit enhanced biological activity against agricultural pests . The development of such intermediates is crucial for creating more effective agrochemicals that minimize environmental impact.
Catalysis Research
Role in Catalytic Reactions:
In catalytic chemistry, this compound has been studied for its potential as a catalyst or catalytic enhancer. Its stannyl groups can influence the rate and selectivity of various catalytic processes.
Experimental Insights:
Studies have shown that this compound can significantly enhance the efficiency of palladium-catalyzed cross-coupling reactions, leading to more efficient reaction pathways . The ability to modify reaction conditions allows for the selective synthesis of desired products.
Nanotechnology
Applications in Nanomaterials Synthesis:
The compound is being investigated for its ability to form stable bonds with other elements at the nanoscale, making it valuable in synthesizing nanocomposites and nanostructures.
Future Directions:
While research is still in its early stages, preliminary findings suggest that this compound could lead to the development of nanomaterials with unique properties suitable for various applications, including electronics and materials science .
Summary of Findings
The applications of this compound span multiple fields of research:
Mechanism of Action
The mechanism of action of 2,5-Bis(tri-n-butylstannyl)furan involves its ability to participate in various chemical reactions due to the presence of the tri-n-butylstannyl groups. These groups can act as leaving groups in substitution reactions or as catalysts in coupling reactions . The molecular targets and pathways involved depend on the specific reaction and application .
Comparison with Similar Compounds
2,5-Bis(tri-n-butylstannyl)furan is unique due to its specific substitution pattern on the furan ring and the presence of two tri-n-butylstannyl groups. Similar compounds include:
2-(Tri-n-butylstannyl)furan: This compound has only one tri-n-butylstannyl group attached to the furan ring.
2,5-Bis(tri-n-butyltin)dibromofuran: This is a product formed from the reaction of this compound with bromine.
These similar compounds share some chemical properties but differ in their reactivity and applications due to the number and position of the stannyl groups .
Biological Activity
2,5-Bis(tri-n-butylstannyl)furan is an organotin compound with significant potential in various fields, particularly in organic synthesis and medicinal chemistry. This article explores its biological activity, mechanisms of action, and applications in drug development, supported by relevant data and findings from diverse sources.
Chemical Structure and Properties
- Molecular Formula : C28H56OSn2
- Molecular Weight : 646.17 g/mol
- Chemical Structure : The compound features a furan ring substituted at the 2 and 5 positions with tri-n-butylstannyl groups, which enhances its reactivity and utility in synthesis.
This compound primarily functions as a reagent in cross-coupling reactions, particularly the Stille coupling reaction. This mechanism allows for the formation of new carbon-carbon bonds, facilitating the synthesis of various biologically active compounds. The stannyl groups serve as nucleophiles that can react with electrophilic partners, leading to the generation of complex molecular architectures.
Antimicrobial Activity
Research indicates that derivatives synthesized from this compound exhibit antimicrobial properties. For instance, compounds formed through Stille coupling with aryl halides have shown activity against various pathogens.
- Case Study : A study demonstrated that coupling this compound with 4-bromobenzonitrile yielded a compound with antimicrobial properties, suggesting its potential as a precursor for developing new antibiotics .
Antiparasitic Activity
The compound has also been investigated for its antiparasitic effects. Certain derivatives have shown promise against parasites such as Trypanosoma brucei and Plasmodium falciparum.
- Research Findings : A study highlighted that modifications to the furan structure could enhance activity against these parasites, indicating that the biological activity is closely tied to structural variations introduced during synthesis .
Applications in Drug Development
This compound serves as a crucial intermediate in synthesizing various pharmaceutical agents:
- Dicationic Bisguanidine-Arylfuran Derivatives : These compounds have been synthesized using this compound as a precursor. The resulting derivatives exhibited significant biological activity and are being explored for therapeutic applications .
- DNA Minor-Groove Binders : Research has shown that derivatives of this compound can act as DNA minor-groove binders, which may lead to new chemotherapeutic agents .
Comparative Analysis
The following table summarizes key findings related to the biological activity of this compound and its derivatives:
Q & A
Basic Research Questions
Q. What are the optimal synthetic routes for preparing 2,5-Bis(tri-n-butylstannyl)furan, and how can side reactions be minimized?
The synthesis typically involves stannylation of furan derivatives. A common approach is the reaction of 2,5-dilithiofuran with tri-n-butyltin chloride under inert conditions. Key parameters include temperature control (−78°C to room temperature), solvent choice (e.g., THF or ethers), and stoichiometric ratios to avoid over-stannylation or decomposition. Side reactions like tin-ligand disproportionation can be mitigated by using freshly distilled reagents and excluding moisture. Characterization via NMR and mass spectrometry is critical to confirm purity .
Q. What spectroscopic methods are most effective for characterizing this compound?
- and NMR : Identify furan ring protons (δ 6.5–7.5 ppm) and tin-bound butyl groups (δ 0.8–1.6 ppm).
- NMR : Confirm tin environments (typical δ −10 to +50 ppm for trialkyltin compounds).
- Mass Spectrometry (EI/ESI) : Detect molecular ion peaks and fragmentation patterns (e.g., loss of butyl groups).
- XRD : Resolve crystal structure to verify stereochemistry and bond angles .
Q. How does the steric bulk of tri-n-butylstannyl groups influence the reactivity of the furan ring?
The bulky tributyltin groups shield the furan ring, reducing electrophilic substitution but enhancing stability toward oxidation. This steric hindrance can direct reactivity toward transmetallation or Stille coupling reactions, where the tin groups act as transfer agents. Comparative studies with less bulky substituents (e.g., trimethyltin) show faster reaction kinetics but lower thermal stability .
Advanced Research Questions
Q. What catalytic systems are effective for Stille coupling reactions involving this compound?
Palladium-based catalysts (e.g., Pd(PPh) or Pd(dba)) are widely used. Key considerations:
- Ligand design : Bulky ligands (e.g., AsPh) improve selectivity by reducing homocoupling.
- Solvent effects : Polar aprotic solvents (DMF, DMSO) enhance solubility but may increase side reactions.
- Additives : CuI or LiCl accelerates transmetallation. Monitor reaction progress via GC-MS to optimize yields .
Q. How do electronic effects of the tin substituents impact the furan ring’s redox behavior?
Cyclic voltammetry studies reveal that electron-donating tri-n-butylstannyl groups lower the oxidation potential of the furan ring compared to unsubstituted furans. This facilitates applications in electrochemical synthesis, such as forming conductive polymers. Computational DFT analysis can model frontier molecular orbitals (HOMO/LUMO) to predict reactivity patterns .
Q. What are the thermal stability and decomposition pathways of this compound under varying conditions?
Thermogravimetric analysis (TGA) shows decomposition onset at ~180°C, releasing butyl fragments and forming tin oxides. In solution, prolonged exposure to light or oxygen accelerates degradation. Stabilizers like BHT (butylated hydroxytoluene) or storage under argon can extend shelf life. LC-MS/MS identifies decomposition byproducts (e.g., dibutyltin oxides) .
Q. Data Contradiction Analysis
Q. How can discrepancies in reported catalytic efficiencies for Stille couplings be resolved?
Contradictions often arise from differences in:
- Catalyst pre-activation : Some protocols pre-reduce Pd catalysts, while others rely on in situ activation.
- Substrate purity : Trace moisture or oxygen degrades tin reagents, skewing results.
- Analytical methods : HPLC vs. NMR integration may yield varying yield calculations. Standardizing reaction conditions and characterization protocols is essential .
Q. Why do some studies report low yields in one-pot syntheses of stannylated furans?
Competing side reactions (e.g., tin-halogen exchange or furan ring hydrogenation) are common. Kinetic studies using in situ IR or Raman spectroscopy can identify intermediate species. Adjusting stoichiometry (e.g., excess Li bases) or switching to milder stannylation agents (e.g., hexaalkyldistannanes) often improves yields .
Q. Methodological Tables
Table 1. Comparison of Catalysts for Stille Coupling
Catalyst | Ligand | Solvent | Yield (%) | Side Products |
---|---|---|---|---|
Pd(PPh) | PPh | THF | 78 | Homocoupled furan |
Pd(dba) | AsPh | DMF | 92 | None |
PdCl(MeCN) | None | Toluene | 65 | Tin oxides |
Table 2. Thermal Stability Under Different Atmospheres
Atmosphere | Decomposition Onset (°C) | Major Byproducts |
---|---|---|
N | 185 | Butyl fragments |
Air | 160 | SnO, CO |
O | 150 | SnO, HO |
Properties
IUPAC Name |
tributyl-(5-tributylstannylfuran-2-yl)stannane | |
---|---|---|
Source | PubChem | |
URL | https://pubchem.ncbi.nlm.nih.gov | |
Description | Data deposited in or computed by PubChem | |
InChI |
InChI=1S/C4H2O.6C4H9.2Sn/c1-2-4-5-3-1;6*1-3-4-2;;/h1-2H;6*1,3-4H2,2H3;; | |
Source | PubChem | |
URL | https://pubchem.ncbi.nlm.nih.gov | |
Description | Data deposited in or computed by PubChem | |
InChI Key |
TWUYAXFJUMLGIN-UHFFFAOYSA-N | |
Source | PubChem | |
URL | https://pubchem.ncbi.nlm.nih.gov | |
Description | Data deposited in or computed by PubChem | |
Canonical SMILES |
CCCC[Sn](CCCC)(CCCC)C1=CC=C(O1)[Sn](CCCC)(CCCC)CCCC | |
Source | PubChem | |
URL | https://pubchem.ncbi.nlm.nih.gov | |
Description | Data deposited in or computed by PubChem | |
Molecular Formula |
C28H56OSn2 | |
Source | PubChem | |
URL | https://pubchem.ncbi.nlm.nih.gov | |
Description | Data deposited in or computed by PubChem | |
DSSTOX Substance ID |
DTXSID10468839 | |
Record name | 2,5-Bis(tri-n-butylstannyl)furan | |
Source | EPA DSSTox | |
URL | https://comptox.epa.gov/dashboard/DTXSID10468839 | |
Description | DSSTox provides a high quality public chemistry resource for supporting improved predictive toxicology. | |
Molecular Weight |
646.2 g/mol | |
Source | PubChem | |
URL | https://pubchem.ncbi.nlm.nih.gov | |
Description | Data deposited in or computed by PubChem | |
CAS No. |
193361-76-1 | |
Record name | 2,5-Bis(tri-n-butylstannyl)furan | |
Source | EPA DSSTox | |
URL | https://comptox.epa.gov/dashboard/DTXSID10468839 | |
Description | DSSTox provides a high quality public chemistry resource for supporting improved predictive toxicology. | |
Retrosynthesis Analysis
AI-Powered Synthesis Planning: Our tool employs the Template_relevance Pistachio, Template_relevance Bkms_metabolic, Template_relevance Pistachio_ringbreaker, Template_relevance Reaxys, Template_relevance Reaxys_biocatalysis model, leveraging a vast database of chemical reactions to predict feasible synthetic routes.
One-Step Synthesis Focus: Specifically designed for one-step synthesis, it provides concise and direct routes for your target compounds, streamlining the synthesis process.
Accurate Predictions: Utilizing the extensive PISTACHIO, BKMS_METABOLIC, PISTACHIO_RINGBREAKER, REAXYS, REAXYS_BIOCATALYSIS database, our tool offers high-accuracy predictions, reflecting the latest in chemical research and data.
Strategy Settings
Precursor scoring | Relevance Heuristic |
---|---|
Min. plausibility | 0.01 |
Model | Template_relevance |
Template Set | Pistachio/Bkms_metabolic/Pistachio_ringbreaker/Reaxys/Reaxys_biocatalysis |
Top-N result to add to graph | 6 |
Feasible Synthetic Routes
Disclaimer and Information on In-Vitro Research Products
Please be aware that all articles and product information presented on BenchChem are intended solely for informational purposes. The products available for purchase on BenchChem are specifically designed for in-vitro studies, which are conducted outside of living organisms. In-vitro studies, derived from the Latin term "in glass," involve experiments performed in controlled laboratory settings using cells or tissues. It is important to note that these products are not categorized as medicines or drugs, and they have not received approval from the FDA for the prevention, treatment, or cure of any medical condition, ailment, or disease. We must emphasize that any form of bodily introduction of these products into humans or animals is strictly prohibited by law. It is essential to adhere to these guidelines to ensure compliance with legal and ethical standards in research and experimentation.