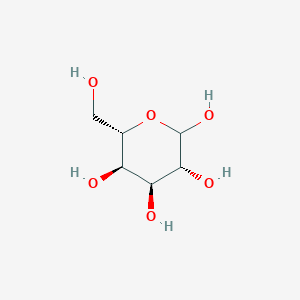
L-Altrose
Overview
Description
L-altropyranose is the pyranose form of L-altrose. It is a this compound and an altropyranose. It is an enantiomer of a D-altropyranose.
Scientific Research Applications
Synthesis and Derivatives
L-Altrose has been explored in various chemical syntheses. For instance, Lunau and Meier (2012) developed a chemical synthesis method for this compound and its 6-deoxy derivative starting from D-galactose and D-fucose. This synthesis involved a Mitsunobu inversion of the open-chain D-hexoses as a key step (Lunau & Meier, 2012). Additionally, Hung et al. (2000, 2001) described convenient routes for the synthesis of this compound from various chemical compounds, highlighting the flexibility and potential of this compound in organic chemistry (Hung et al., 2000); (Hung et al., 2001).
Polysaccharides and Bacterial Lipopolysaccharides
This compound has been identified in various natural sources and is significant in bacterial polysaccharides. Tako et al. (2012) identified 6-deoxy-D-altrose, an isomer of this compound, in an edible mushroom, which was a novel discovery in the natural environment (Tako et al., 2012). Stack and Ericsson (1988) developed methods for detecting and analyzing this compound in bacterial polysaccharides, which is crucial for understanding its role in bacterial structures (Stack & Ericsson, 1988).
Role in Bacterial Surface Glycans
Andolina et al. (2018) studied the role of this compound in bacterial surface glycans, particularly in the context of antibiotic-resistant bacteria. They developed a synthesis for derivatives of this compound to explore its biological significance and potential in combating drug-resistant bacterial infections (Andolina et al., 2018).
Enzymatic Production
Menavuvu et al. (2006) reported the novel substrate specificity of D-arabinose isomerase, which included this compound. This study showed the potential for enzymatic production of this compound from other sugars, which has implications for both biochemical research and industrial applications (Menavuvu et al., 2006).
Agricultural Implications
Kano et al. (2010) studied the effects of rare sugars, including D-altrose, on rice plants. They found that D-allose, a sugar related to D-altrose, had an inhibitory effect on growth but conferred resistance to bacterial blight, indicating potential agricultural applications of related sugars (Kano et al., 2010).
properties
Molecular Formula |
C6H12O6 |
---|---|
Molecular Weight |
180.16 g/mol |
IUPAC Name |
(3R,4S,5R,6S)-6-(hydroxymethyl)oxane-2,3,4,5-tetrol |
InChI |
InChI=1S/C6H12O6/c7-1-2-3(8)4(9)5(10)6(11)12-2/h2-11H,1H2/t2-,3-,4-,5+,6?/m0/s1 |
InChI Key |
WQZGKKKJIJFFOK-VSOAQEOCSA-N |
Isomeric SMILES |
C([C@H]1[C@@H]([C@@H]([C@H](C(O1)O)O)O)O)O |
SMILES |
C(C1C(C(C(C(O1)O)O)O)O)O |
Canonical SMILES |
C(C1C(C(C(C(O1)O)O)O)O)O |
Origin of Product |
United States |
Synthesis routes and methods I
Procedure details
Synthesis routes and methods II
Procedure details
Disclaimer and Information on In-Vitro Research Products
Please be aware that all articles and product information presented on BenchChem are intended solely for informational purposes. The products available for purchase on BenchChem are specifically designed for in-vitro studies, which are conducted outside of living organisms. In-vitro studies, derived from the Latin term "in glass," involve experiments performed in controlled laboratory settings using cells or tissues. It is important to note that these products are not categorized as medicines or drugs, and they have not received approval from the FDA for the prevention, treatment, or cure of any medical condition, ailment, or disease. We must emphasize that any form of bodily introduction of these products into humans or animals is strictly prohibited by law. It is essential to adhere to these guidelines to ensure compliance with legal and ethical standards in research and experimentation.