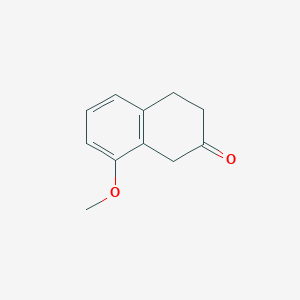
8-Methoxy-2-tetralone
Overview
Description
8-Methoxy-2-tetralone: is an organic compound with the molecular formula C11H12O2 . It is a derivative of tetralone, characterized by a methoxy group at the 8th position of the tetralone structure.
Mechanism of Action
Target of Action
This compound is a derivative of tetralone, a class of compounds that have been studied for their potential biological activities . .
Biochemical Pathways
The biochemical pathways influenced by 8-Methoxy-2-tetralone are not well-defined. As a derivative of tetralone, it may potentially influence similar biochemical pathways as other tetralone compounds. Without specific studies on this compound, it is difficult to definitively state which biochemical pathways it affects .
Pharmacokinetics
The compound has a molecular weight of 176.21 , which suggests it may have favorable bioavailability due to its relatively small size.
Result of Action
The molecular and cellular effects of this compound are currently unknown. While some tetralone derivatives have been studied for their potential biological activities
Action Environment
The influence of environmental factors on the action, efficacy, and stability of this compound is not well-understood. Factors such as temperature, pH, and the presence of other molecules can potentially influence the action of a compound. Without specific studies, it is difficult to determine how these factors might influence this compound .
Preparation Methods
Synthetic Routes and Reaction Conditions: The synthesis of 8-Methoxy-2-tetralone typically involves the following steps:
Friedel-Crafts Acylation: Anisole is reacted with succinic anhydride in the presence of aluminum chloride to form 4-(4-methoxyphenyl)-4-oxobutanoic acid.
Reduction: The resulting compound is then reduced to 4-(4-methoxyphenyl)butanoic acid.
Cyclization: The final step involves cyclization using methanesulfonic acid to yield this compound.
Industrial Production Methods: In industrial settings, continuous-flow synthesis is often employed to enhance reaction efficiency and yield. This method allows for better control over reaction conditions and reduces reaction time significantly compared to traditional batch processes .
Chemical Reactions Analysis
Types of Reactions: 8-Methoxy-2-tetralone undergoes various chemical reactions, including:
Oxidation: It can be oxidized to form corresponding quinones.
Reduction: Reduction reactions can convert it into 8-methoxy-2-tetralol.
Substitution: Electrophilic substitution reactions can introduce different substituents on the aromatic ring.
Common Reagents and Conditions:
Oxidation: Common oxidizing agents include potassium permanganate and chromium trioxide.
Reduction: Sodium borohydride or lithium aluminum hydride are typically used for reduction reactions.
Substitution: Friedel-Crafts acylation or alkylation reactions often use aluminum chloride as a catalyst.
Major Products:
Oxidation: Produces quinones.
Reduction: Yields 8-methoxy-2-tetralol.
Substitution: Results in various substituted tetralones depending on the reagents used.
Scientific Research Applications
8-Methoxy-2-tetralone has several applications in scientific research:
Chemistry: It is used as a building block in the synthesis of more complex organic molecules.
Biology: It serves as a precursor in the synthesis of biologically active compounds, including potential pharmaceuticals.
Medicine: Research has explored its use in the development of drugs targeting central nervous system disorders.
Industry: It is utilized in the production of materials with specific chemical properties
Comparison with Similar Compounds
- 6-Methoxy-1-tetralone
- 7-Methoxy-1-tetralone
- 2,6-Dihydroxynaphthalene
- 1,6-Dihydroxynaphthalene
Comparison: 8-Methoxy-2-tetralone is unique due to the position of the methoxy group, which influences its chemical reactivity and biological activity. Compared to
Properties
IUPAC Name |
8-methoxy-3,4-dihydro-1H-naphthalen-2-one | |
---|---|---|
Source | PubChem | |
URL | https://pubchem.ncbi.nlm.nih.gov | |
Description | Data deposited in or computed by PubChem | |
InChI |
InChI=1S/C11H12O2/c1-13-11-4-2-3-8-5-6-9(12)7-10(8)11/h2-4H,5-7H2,1H3 | |
Source | PubChem | |
URL | https://pubchem.ncbi.nlm.nih.gov | |
Description | Data deposited in or computed by PubChem | |
InChI Key |
BTYBORAHYUCUMH-UHFFFAOYSA-N | |
Source | PubChem | |
URL | https://pubchem.ncbi.nlm.nih.gov | |
Description | Data deposited in or computed by PubChem | |
Canonical SMILES |
COC1=CC=CC2=C1CC(=O)CC2 | |
Source | PubChem | |
URL | https://pubchem.ncbi.nlm.nih.gov | |
Description | Data deposited in or computed by PubChem | |
Molecular Formula |
C11H12O2 | |
Source | PubChem | |
URL | https://pubchem.ncbi.nlm.nih.gov | |
Description | Data deposited in or computed by PubChem | |
DSSTOX Substance ID |
DTXSID20399746 | |
Record name | 8-Methoxy-2-tetralone | |
Source | EPA DSSTox | |
URL | https://comptox.epa.gov/dashboard/DTXSID20399746 | |
Description | DSSTox provides a high quality public chemistry resource for supporting improved predictive toxicology. | |
Molecular Weight |
176.21 g/mol | |
Source | PubChem | |
URL | https://pubchem.ncbi.nlm.nih.gov | |
Description | Data deposited in or computed by PubChem | |
CAS No. |
5309-19-3 | |
Record name | 8-Methoxy-2-tetralone | |
Source | EPA DSSTox | |
URL | https://comptox.epa.gov/dashboard/DTXSID20399746 | |
Description | DSSTox provides a high quality public chemistry resource for supporting improved predictive toxicology. | |
Record name | 8-Methoxy-2-tetralone | |
Source | European Chemicals Agency (ECHA) | |
URL | https://echa.europa.eu/information-on-chemicals | |
Description | The European Chemicals Agency (ECHA) is an agency of the European Union which is the driving force among regulatory authorities in implementing the EU's groundbreaking chemicals legislation for the benefit of human health and the environment as well as for innovation and competitiveness. | |
Explanation | Use of the information, documents and data from the ECHA website is subject to the terms and conditions of this Legal Notice, and subject to other binding limitations provided for under applicable law, the information, documents and data made available on the ECHA website may be reproduced, distributed and/or used, totally or in part, for non-commercial purposes provided that ECHA is acknowledged as the source: "Source: European Chemicals Agency, http://echa.europa.eu/". Such acknowledgement must be included in each copy of the material. ECHA permits and encourages organisations and individuals to create links to the ECHA website under the following cumulative conditions: Links can only be made to webpages that provide a link to the Legal Notice page. | |
Retrosynthesis Analysis
AI-Powered Synthesis Planning: Our tool employs the Template_relevance Pistachio, Template_relevance Bkms_metabolic, Template_relevance Pistachio_ringbreaker, Template_relevance Reaxys, Template_relevance Reaxys_biocatalysis model, leveraging a vast database of chemical reactions to predict feasible synthetic routes.
One-Step Synthesis Focus: Specifically designed for one-step synthesis, it provides concise and direct routes for your target compounds, streamlining the synthesis process.
Accurate Predictions: Utilizing the extensive PISTACHIO, BKMS_METABOLIC, PISTACHIO_RINGBREAKER, REAXYS, REAXYS_BIOCATALYSIS database, our tool offers high-accuracy predictions, reflecting the latest in chemical research and data.
Strategy Settings
Precursor scoring | Relevance Heuristic |
---|---|
Min. plausibility | 0.01 |
Model | Template_relevance |
Template Set | Pistachio/Bkms_metabolic/Pistachio_ringbreaker/Reaxys/Reaxys_biocatalysis |
Top-N result to add to graph | 6 |
Feasible Synthetic Routes
Q1: What is the significance of 8-Methoxy-2-tetralone in organic synthesis?
A1: this compound serves as a versatile building block in organic synthesis, particularly in the construction of complex polycyclic structures. For example, it acts as a crucial starting material in synthesizing various derivatives of 7H-dibenzo[c,g]carbazole, a potent carcinogen. This compound also finds utility in the development of potential pharmaceuticals, as evidenced by its role in synthesizing radiolabeled ligands for studying serotonin receptors.
Q2: Can you describe an efficient synthesis route for this compound?
A2: A streamlined synthesis of this compound starts with 2-bromophenylacetic acid and achieves an overall yield of approximately 50%. The key steps involve a Friedel-Crafts acylation/cyclization, ketone protection, a copper(I)-catalyzed methoxylation of the aromatic bromide (using an ester as a co-catalyst), and finally, ketone deprotection.
Q3: How is this compound utilized in preparing biologically active compounds?
A3: this compound plays a crucial role in the multi-step synthesis of R‐(+)‐ and S‐(−)‐8‐hydroxy‐2‐(N, N‐dipropylamino)-[2‐3H] tetralin. HCl (8‐OH‐DPAT), a 5HT1A receptor agonist. The process involves reductive amination, acylation, reduction, and chiral separation to yield the target compound.
Q4: How is this compound utilized in studying gibberellin synthesis?
A4: Researchers utilize this compound to synthesize intermediates containing the cyclohexa-2,4-dienone moiety, relevant for gibberellin synthesis. For instance, it can be converted to 1,2,3,4-tetrahydro-8-hydroxy-2-naphthoic acid, which upon diazotization and acid-catalyzed reaction, yields tricyclic ketones with the desired cyclohexa-2,4-dienone structure.
Disclaimer and Information on In-Vitro Research Products
Please be aware that all articles and product information presented on BenchChem are intended solely for informational purposes. The products available for purchase on BenchChem are specifically designed for in-vitro studies, which are conducted outside of living organisms. In-vitro studies, derived from the Latin term "in glass," involve experiments performed in controlled laboratory settings using cells or tissues. It is important to note that these products are not categorized as medicines or drugs, and they have not received approval from the FDA for the prevention, treatment, or cure of any medical condition, ailment, or disease. We must emphasize that any form of bodily introduction of these products into humans or animals is strictly prohibited by law. It is essential to adhere to these guidelines to ensure compliance with legal and ethical standards in research and experimentation.