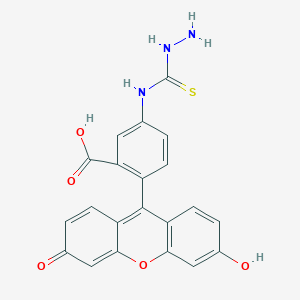
Fluorescein-5-thiosemicarbazide
Overview
Description
Fluorescein-5-thiosemicarbazide is a fluorescent probe widely used in various scientific fields. It is known for its strong fluorescence properties and compatibility with living cells, making it an essential tool in biological imaging and molecular studies .
Mechanism of Action
Target of Action
Fluorescein-5-thiosemicarbazide (FTSC) primarily targets mucin-type O-linked glycoproteins within living cells . These glycoproteins play a significant role in regulating many aspects of cell activity .
Mode of Action
FTSC interacts with its targets through a direct labeling strategy developed for in situ visualization of mucin-type O-linked glycoproteins on living cells . This strategy involves treating cells with an unnatural sugar, 2-keto Ac4GalNAc analogue (2-keto isostere of GalNAc), to generate keto groups upon cells . This is followed by chemoselective ligation of keto groups on cells with FTSC, which serves as a fluorescent tag .
Biochemical Pathways
The biochemical pathway affected by FTSC involves O-glycosylation , a process initiated in the Golgi compartment by a family of polypeptide N-acetyl-α-galactosaminyl transferases . The interaction of FTSC with its targets leads to changes in this pathway, enabling the visualization of mucin-type O-linked glycoproteins .
Pharmacokinetics
It’s known that cells treated with ftsc maintain a high level of viability , suggesting that the compound may have favorable bioavailability and minimal toxicity.
Result of Action
The primary result of FTSC’s action is the successful imaging of the expression of mucin-type O-linked glycoproteins within living cells . This is achieved through the fluorescent spectra of FTSC conjugates, which are similar to FITC, a fluorescent tag widely used in proteomics .
Action Environment
The action of FTSC is influenced by the cellular environment, particularly the presence of keto groups generated by treating cells with the 2-keto Ac4GalNAc analogue . The fluorescent properties of FTSC, including its excitation and emission wavelengths, are compatible with commonly used fluorescent equipment , suggesting that the compound’s action, efficacy, and stability are well-suited to standard laboratory conditions.
Biochemical Analysis
Biochemical Properties
Fluorescein-5-thiosemicarbazide plays a crucial role in biochemical reactions, particularly in the labeling of cell-surface functional groups such as glycophorins. It interacts with aldehydes and ketones to form stable conjugates, which can then be visualized using fluorescence microscopy. This compound is compatible with commonly used fluorescent equipment, making it a versatile tool for studying various biochemical processes. This compound has been shown to interact with mucin-type O-linked glycoproteins, which are involved in regulating many aspects of cell activity .
Cellular Effects
This compound has been used to study the effects of various compounds on cell function. It has been employed to label and image mucin-type O-linked glycoproteins within living cells, providing insights into their role in cellular processes. The compound has been shown to maintain a high level of cell viability, with treated cells exhibiting a viability of 84.3% . This indicates that this compound is relatively non-toxic and can be used for long-term imaging studies without significantly affecting cell function.
Molecular Mechanism
At the molecular level, this compound exerts its effects through the formation of stable conjugates with aldehydes and ketones. This reaction is facilitated by the intrinsic reactivity of the thiosemicarbazide group towards these functional groups. The resulting conjugates exhibit strong fluorescence properties, allowing for the visualization of labeled biomolecules. This mechanism has been utilized to label various biomolecules, including glycophorins and mucin-type O-linked glycoproteins, enabling the study of their distribution and interactions within cells .
Temporal Effects in Laboratory Settings
In laboratory settings, the effects of this compound have been observed to change over time. The compound is relatively stable under physiological conditions, maintaining its fluorescence properties for extended periods. The stability of the conjugates formed with aldehydes and ketones can vary depending on the specific biomolecule being labeled. Long-term studies have shown that this compound can be used for continuous imaging of cellular processes without significant degradation .
Dosage Effects in Animal Models
The effects of this compound in animal models have been studied to determine its safety and efficacy at different dosages. Studies have shown that the compound is relatively non-toxic at low to moderate doses, with minimal adverse effects observed. At high doses, this compound can exhibit toxic effects, including reduced cell viability and altered cellular function. These findings highlight the importance of optimizing the dosage to achieve the desired labeling and imaging effects while minimizing potential toxicity .
Metabolic Pathways
This compound is involved in metabolic pathways related to the labeling and imaging of biomolecules. It interacts with enzymes and cofactors involved in the formation of aldehydes and ketones, facilitating the formation of stable conjugates. This interaction can affect metabolic flux and metabolite levels, providing insights into the metabolic processes within cells. The compound has been used to study the metabolism of various biomolecules, including glycophorins and mucin-type O-linked glycoproteins .
Transport and Distribution
Within cells and tissues, this compound is transported and distributed through interactions with transporters and binding proteins. These interactions facilitate the localization and accumulation of the compound in specific cellular compartments. The distribution of this compound can be visualized using fluorescence microscopy, providing insights into its transport and localization within cells. This information is valuable for understanding the dynamics of cellular processes and the role of specific biomolecules .
Subcellular Localization
This compound exhibits specific subcellular localization patterns, which can affect its activity and function. The compound is often directed to specific compartments or organelles through targeting signals or post-translational modifications. This localization can influence the interactions of this compound with biomolecules and its overall effectiveness as a labeling and imaging tool. Studies have shown that the compound can be used to visualize the distribution of mucin-type O-linked glycoproteins within various subcellular compartments .
Preparation Methods
Synthetic Routes and Reaction Conditions: Fluorescein-5-thiosemicarbazide is synthesized through the reaction of fluorescein isothiocyanate with thiosemicarbazide under mild and neutral conditions. This reaction avoids the formation of lactone byproducts, ensuring high yield and purity .
Industrial Production Methods: The industrial production of this compound involves large-scale synthesis using similar reaction conditions. The process is optimized to ensure high efficiency and minimal waste, making it suitable for commercial applications .
Chemical Reactions Analysis
Types of Reactions: Fluorescein-5-thiosemicarbazide undergoes various chemical reactions, including:
Oxidation: Reacts with oxidizing agents to form oxidized products.
Reduction: Can be reduced under specific conditions to yield reduced forms.
Substitution: Participates in substitution reactions with aldehydes and ketones to form thiosemicarbazones.
Common Reagents and Conditions:
Oxidation: Common oxidizing agents include hydrogen peroxide and potassium permanganate.
Reduction: Reducing agents such as sodium borohydride are used.
Substitution: Reactions with aldehydes and ketones are typically carried out in aqueous or organic solvents under mild conditions.
Major Products:
Oxidation: Oxidized fluorescein derivatives.
Reduction: Reduced fluorescein derivatives.
Substitution: Thiosemicarbazones.
Scientific Research Applications
Fluorescein-5-thiosemicarbazide has a wide range of applications in scientific research, including:
Chemistry: Used as a fluorescent tag for labeling various molecules.
Biology: Employed in imaging studies to visualize cellular processes and structures.
Medicine: Utilized in diagnostic assays and drug delivery systems.
Industry: Applied in the development of fluorescent sensors and probes for various industrial processes
Comparison with Similar Compounds
Fluorescein isothiocyanate: Another fluorescent probe with similar spectral properties.
Rhodamine B: A fluorescent dye used in similar applications.
Texas Red: A red fluorescent dye with applications in biological imaging.
Uniqueness: Fluorescein-5-thiosemicarbazide is unique due to its strong fluorescence, compatibility with living cells, and specific reactivity with aldehyde groups. These properties make it a preferred choice for various scientific applications .
Properties
IUPAC Name |
5-(aminocarbamothioylamino)-2-(3-hydroxy-6-oxoxanthen-9-yl)benzoic acid | |
---|---|---|
Source | PubChem | |
URL | https://pubchem.ncbi.nlm.nih.gov | |
Description | Data deposited in or computed by PubChem | |
InChI |
InChI=1S/C21H15N3O5S/c22-24-21(30)23-10-1-4-13(16(7-10)20(27)28)19-14-5-2-11(25)8-17(14)29-18-9-12(26)3-6-15(18)19/h1-9,25H,22H2,(H,27,28)(H2,23,24,30) | |
Source | PubChem | |
URL | https://pubchem.ncbi.nlm.nih.gov | |
Description | Data deposited in or computed by PubChem | |
InChI Key |
MEUCHQDLZYLNQY-UHFFFAOYSA-N | |
Source | PubChem | |
URL | https://pubchem.ncbi.nlm.nih.gov | |
Description | Data deposited in or computed by PubChem | |
Canonical SMILES |
C1=CC(=C(C=C1NC(=S)NN)C(=O)O)C2=C3C=CC(=O)C=C3OC4=C2C=CC(=C4)O | |
Source | PubChem | |
URL | https://pubchem.ncbi.nlm.nih.gov | |
Description | Data deposited in or computed by PubChem | |
Molecular Formula |
C21H15N3O5S | |
Source | PubChem | |
URL | https://pubchem.ncbi.nlm.nih.gov | |
Description | Data deposited in or computed by PubChem | |
DSSTOX Substance ID |
DTXSID60397968 | |
Record name | Fluorescein-5-thiosemicarbazide | |
Source | EPA DSSTox | |
URL | https://comptox.epa.gov/dashboard/DTXSID60397968 | |
Description | DSSTox provides a high quality public chemistry resource for supporting improved predictive toxicology. | |
Molecular Weight |
421.4 g/mol | |
Source | PubChem | |
URL | https://pubchem.ncbi.nlm.nih.gov | |
Description | Data deposited in or computed by PubChem | |
CAS No. |
76863-28-0 | |
Record name | Fluorescein-5-thiosemicarbazide | |
Source | EPA DSSTox | |
URL | https://comptox.epa.gov/dashboard/DTXSID60397968 | |
Description | DSSTox provides a high quality public chemistry resource for supporting improved predictive toxicology. | |
Retrosynthesis Analysis
AI-Powered Synthesis Planning: Our tool employs the Template_relevance Pistachio, Template_relevance Bkms_metabolic, Template_relevance Pistachio_ringbreaker, Template_relevance Reaxys, Template_relevance Reaxys_biocatalysis model, leveraging a vast database of chemical reactions to predict feasible synthetic routes.
One-Step Synthesis Focus: Specifically designed for one-step synthesis, it provides concise and direct routes for your target compounds, streamlining the synthesis process.
Accurate Predictions: Utilizing the extensive PISTACHIO, BKMS_METABOLIC, PISTACHIO_RINGBREAKER, REAXYS, REAXYS_BIOCATALYSIS database, our tool offers high-accuracy predictions, reflecting the latest in chemical research and data.
Strategy Settings
Precursor scoring | Relevance Heuristic |
---|---|
Min. plausibility | 0.01 |
Model | Template_relevance |
Template Set | Pistachio/Bkms_metabolic/Pistachio_ringbreaker/Reaxys/Reaxys_biocatalysis |
Top-N result to add to graph | 6 |
Feasible Synthetic Routes
Q1: How does Fluorescein-5-thiosemicarbazide interact with its target?
A1: this compound reacts specifically with carbonyl groups (aldehydes and ketones) present in biomolecules like proteins and carbohydrates. This reaction forms a stable hydrazone bond, covalently linking FTSC to its target [, , ].
Q2: What are the downstream effects of this interaction?
A2: The covalent attachment of FTSC to carbonyl groups allows for:
- Visualization: The fluorescent properties of FTSC enable the detection and imaging of target molecules using techniques like fluorescence microscopy and gel electrophoresis [, , , , ].
- Quantification: FTSC labeling facilitates the quantification of carbonyl groups in biological samples, serving as a marker for oxidative stress [, , ].
- Targeted delivery: FTSC can be conjugated to nanoparticles or other carriers, and its target specificity can be further enhanced by conjugating it with molecules like folate [, , ]. This enables targeted delivery of these carriers to specific cell types, such as cancer cells overexpressing folate receptors [].
Q3: What is the molecular formula and weight of this compound?
A3: The molecular formula of FTSC is C21H17N3O5S, and its molecular weight is 423.44 g/mol [].
Q4: How does the performance and application of FTSC vary under different conditions?
A5: FTSC's performance is influenced by factors like solvent polarity, pH, and temperature. For instance, its quantum yield is notably low in unmodified forms across several solvents []. Additionally, studies employing FTSC for protein labeling often involve steps to optimize reaction conditions, such as pH and reaction time, to ensure effective labeling and minimize background signal [, ].
Q5: Does this compound possess any catalytic properties?
A5: Based on the provided research, FTSC is primarily utilized as a labeling reagent due to its specific reactivity with carbonyl groups and its fluorescent properties. There is no indication of inherent catalytic activity for FTSC in these studies.
Q6: Have computational chemistry approaches been applied to study this compound?
A7: While the provided research doesn't explicitly detail computational studies on FTSC itself, they highlight its use in techniques like 2DE-LC-MS/MS for identifying and quantifying carbonylated proteins [, ]. These approaches often leverage computational tools for data analysis, protein identification, and quantification.
Disclaimer and Information on In-Vitro Research Products
Please be aware that all articles and product information presented on BenchChem are intended solely for informational purposes. The products available for purchase on BenchChem are specifically designed for in-vitro studies, which are conducted outside of living organisms. In-vitro studies, derived from the Latin term "in glass," involve experiments performed in controlled laboratory settings using cells or tissues. It is important to note that these products are not categorized as medicines or drugs, and they have not received approval from the FDA for the prevention, treatment, or cure of any medical condition, ailment, or disease. We must emphasize that any form of bodily introduction of these products into humans or animals is strictly prohibited by law. It is essential to adhere to these guidelines to ensure compliance with legal and ethical standards in research and experimentation.