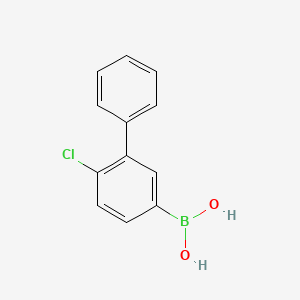
6-Chlorobiphenyl-3-boronic Acid
- Click on QUICK INQUIRY to receive a quote from our team of experts.
- With the quality product at a COMPETITIVE price, you can focus more on your research.
Overview
Description
6-Chlorobiphenyl-3-boronic Acid is an organoboron compound that features a biphenyl structure with a chlorine atom at the 6th position and a boronic acid group at the 3rd position. This compound is particularly significant in organic synthesis, especially in Suzuki-Miyaura cross-coupling reactions, which are widely used for forming carbon-carbon bonds in the synthesis of various organic molecules .
Preparation Methods
Synthetic Routes and Reaction Conditions
The primary method for synthesizing boronic acids, including 6-Chlorobiphenyl-3-boronic Acid, involves the electrophilic trapping of an organometallic reagent with a boric ester, such as trimethyl borate (B(OMe)3) or triisopropyl borate (B(Oi-Pr)3). This reaction is typically performed at low temperatures to prevent over-alkylation, which could lead to the formation of borinic esters instead of boronic acids .
Industrial Production Methods
In industrial settings, the synthesis of this compound may involve large-scale Suzuki-Miyaura coupling reactions. These reactions are conducted under mild and functional group-tolerant conditions, making them suitable for producing significant quantities of the compound .
Chemical Reactions Analysis
Types of Reactions
6-Chlorobiphenyl-3-boronic Acid primarily undergoes substitution reactions, particularly in Suzuki-Miyaura cross-coupling reactions. These reactions involve the formation of carbon-carbon bonds between the boronic acid and an aryl or vinyl halide in the presence of a palladium catalyst .
Common Reagents and Conditions
Reagents: Palladium catalysts (e.g., Pd(PPh3)4), bases (e.g., K2CO3, NaOH), and solvents (e.g., toluene, ethanol).
Major Products
The major products formed from these reactions are biaryl compounds, which are essential intermediates in the synthesis of pharmaceuticals, agrochemicals, and organic materials .
Scientific Research Applications
6-Chlorobiphenyl-3-boronic Acid has diverse applications in scientific research:
Chemistry: Used in the synthesis of complex organic molecules through Suzuki-Miyaura coupling reactions.
Biology: Employed in the development of biochemical tools for molecular recognition and sensing applications.
Industry: Utilized in the production of advanced materials, including polymers and electronic devices.
Mechanism of Action
The mechanism of action of 6-Chlorobiphenyl-3-boronic Acid in Suzuki-Miyaura coupling involves several key steps:
Oxidative Addition: The palladium catalyst undergoes oxidative addition with the aryl or vinyl halide, forming a palladium complex.
Transmetalation: The boronic acid transfers its organic group to the palladium complex.
Reductive Elimination: The palladium complex undergoes reductive elimination, forming the desired biaryl product and regenerating the palladium catalyst.
Comparison with Similar Compounds
Similar Compounds
3-Formylphenylboronic Acid: Another boronic acid derivative used in organic synthesis.
4-Formylphenylboronic Acid: Similar to 3-Formylphenylboronic Acid but with the formyl group at the 4th position.
Phenylboronic Acid: A simpler boronic acid used in various chemical reactions.
Uniqueness
6-Chlorobiphenyl-3-boronic Acid is unique due to its specific substitution pattern, which imparts distinct reactivity and selectivity in cross-coupling reactions. The presence of the chlorine atom at the 6th position can influence the electronic properties of the compound, making it particularly useful in certain synthetic applications .
Properties
Molecular Formula |
C12H10BClO2 |
---|---|
Molecular Weight |
232.47 g/mol |
IUPAC Name |
(4-chloro-3-phenylphenyl)boronic acid |
InChI |
InChI=1S/C12H10BClO2/c14-12-7-6-10(13(15)16)8-11(12)9-4-2-1-3-5-9/h1-8,15-16H |
InChI Key |
AXRKIJHKUZRNIX-UHFFFAOYSA-N |
Canonical SMILES |
B(C1=CC(=C(C=C1)Cl)C2=CC=CC=C2)(O)O |
Origin of Product |
United States |
Disclaimer and Information on In-Vitro Research Products
Please be aware that all articles and product information presented on BenchChem are intended solely for informational purposes. The products available for purchase on BenchChem are specifically designed for in-vitro studies, which are conducted outside of living organisms. In-vitro studies, derived from the Latin term "in glass," involve experiments performed in controlled laboratory settings using cells or tissues. It is important to note that these products are not categorized as medicines or drugs, and they have not received approval from the FDA for the prevention, treatment, or cure of any medical condition, ailment, or disease. We must emphasize that any form of bodily introduction of these products into humans or animals is strictly prohibited by law. It is essential to adhere to these guidelines to ensure compliance with legal and ethical standards in research and experimentation.