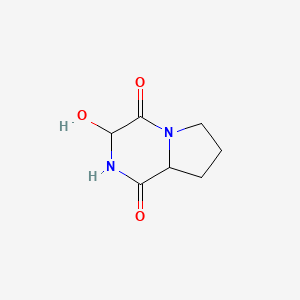
Vildagliptin impurity A-F
- Click on QUICK INQUIRY to receive a quote from our team of experts.
- With the quality product at a COMPETITIVE price, you can focus more on your research.
Overview
Description
Vildagliptin impurity A-F are by-products or degradation products associated with the synthesis and stability of Vildagliptin, a dipeptidyl peptidase-4 (DPP-4) inhibitor used in the treatment of type 2 diabetes mellitus. These impurities are crucial for quality control and regulatory compliance in pharmaceutical manufacturing.
Preparation Methods
Synthetic Routes and Reaction Conditions
The synthesis of Vildagliptin impurities typically involves multiple steps starting from L-proline. For example, one method includes reacting methyl L-prolinate with chloroacetyl chloride under alkaline conditions to generate an intermediate compound. This intermediate then undergoes condensation with 3-amino-1-hydroxyadamantane, followed by a self-condensation reaction under alkaline conditions to yield the impurity .
Industrial Production Methods
Industrial production of Vildagliptin and its impurities involves optimized processes to ensure high yield and purity. These methods often include advanced techniques such as high-performance liquid chromatography (HPLC) for purification and quality control .
Chemical Reactions Analysis
Types of Reactions
Vildagliptin impurities can undergo various chemical reactions, including:
Oxidation: Exposure to oxidizing agents can lead to the formation of oxidized impurities.
Reduction: Reducing agents can convert certain functional groups within the impurities.
Substitution: Halogenation or other substitution reactions can modify the impurity structure.
Common Reagents and Conditions
Common reagents used in these reactions include chloroacetyl chloride for acylation, and various oxidizing and reducing agents depending on the desired transformation. Reaction conditions typically involve controlled temperatures and pH levels to ensure specificity and yield .
Major Products Formed
The major products formed from these reactions are structurally related to Vildagliptin but may include additional functional groups or altered configurations, which are identified and quantified using analytical techniques like HPLC .
Scientific Research Applications
Vildagliptin impurities have several scientific research applications:
Chemistry: Used as reference standards in analytical chemistry to ensure the purity of Vildagliptin.
Biology: Studied for their biological activity and potential effects on metabolic pathways.
Medicine: Evaluated for their safety and efficacy as part of the drug development process.
Industry: Utilized in quality control processes to maintain the integrity of pharmaceutical products.
Mechanism of Action
The mechanism of action for Vildagliptin impurities involves their interaction with the DPP-4 enzyme, similar to Vildagliptin. By inhibiting DPP-4, these impurities can affect the levels of incretin hormones, glucagon-like peptide-1 (GLP-1), and glucose-dependent insulinotropic polypeptide (GIP), thereby influencing glucose metabolism .
Comparison with Similar Compounds
Similar Compounds
Similar compounds to Vildagliptin include other DPP-4 inhibitors such as:
- Sitagliptin
- Saxagliptin
- Alogliptin
- Linagliptin
Uniqueness
Vildagliptin impurities are unique in their specific structural modifications, which can influence their pharmacokinetic and pharmacodynamic properties. These differences are critical for understanding the safety and efficacy of the parent drug .
Biological Activity
Vildagliptin impurity A-F, chemically known as 3-Hydroxyhexahydropyrrolo[1,2-a]pyrazine-1,4-dione, is a notable impurity associated with the oral antihyperglycemic agent Vildagliptin, which is primarily used in the management of type 2 diabetes mellitus. Vildagliptin acts as a dipeptidyl peptidase-4 (DPP-4) inhibitor, enhancing incretin hormone levels to regulate blood glucose. The biological activity of this compound is critical for understanding its role in pharmaceutical formulations, potential toxicity, and interactions with other compounds.
This compound has the molecular formula C7H10N2O3 and a molecular weight of 170.17 g/mol. It can be synthesized through various chemical pathways involving the manipulation of pyrrolidine and pyrazine derivatives. Common synthetic methods include:
- Cyclization reactions : Utilizing pyrrolidine derivatives.
- Hydroxylation : Introducing hydroxyl groups to alter biological activity.
Comparison of Related Compounds
Compound Name | Molecular Formula | Key Features |
---|---|---|
Vildagliptin | C17H25N3O2 | Main active ingredient; DPP-4 inhibitor |
Vildagliptin Amide Impurity | C17H27N3O3 | Contains amide functional group |
Vildagliptin Carboxylic Acid Impurity | C17H25N3O4 | Contains carboxylic acid functional group |
(8aS)-3-Hydroxyhexahydropyrrolo[1,2-a]pyrazine-1,4-dione | C7H10N2O3 | Structural isomer; similar biological activity |
This compound is unique due to its specific hydroxylated structure and lower molecular weight compared to its parent compound and other impurities, which may influence its biological activity differently.
Pharmacological Effects
This compound's biological activity is primarily characterized by its interactions with DPP-4 enzymes, similar to Vildagliptin. However, studies have shown that impurities can significantly affect the pharmacokinetics and pharmacodynamics of the main drug.
Case Studies on Toxicity
- In Vitro Toxic Evaluation : A study assessed the cytotoxic effects of various gliptins and their impurities. It was found that at concentrations of 10 µM, impurities such as V1 and V2 increased oxidative stress without directly causing cytotoxicity in 3T3 cells. The study highlighted that while Vildagliptin itself did not show significant toxicity, the presence of impurities could enhance oxidative stress levels .
- Stability Studies : Research on the stability of Vildagliptin under different conditions revealed that degradation products, including impurities A-F, could form under acidic or oxidative stress conditions. This degradation was quantified using techniques like UHPLC-DAD-MS . The implications for patient safety and drug efficacy are significant as these impurities may alter therapeutic outcomes.
Interaction Studies
Interaction studies involving this compound focus on its effects when co-administered with other medications or compounds. These studies are crucial for understanding potential drug-drug interactions that could alter pharmacokinetics or pharmacodynamics .
Properties
Molecular Formula |
C7H10N2O3 |
---|---|
Molecular Weight |
170.17 g/mol |
IUPAC Name |
3-hydroxy-2,3,6,7,8,8a-hexahydropyrrolo[1,2-a]pyrazine-1,4-dione |
InChI |
InChI=1S/C7H10N2O3/c10-5-4-2-1-3-9(4)7(12)6(11)8-5/h4,6,11H,1-3H2,(H,8,10) |
InChI Key |
GVQWXLTWWOSRCG-UHFFFAOYSA-N |
Canonical SMILES |
C1CC2C(=O)NC(C(=O)N2C1)O |
Origin of Product |
United States |
Disclaimer and Information on In-Vitro Research Products
Please be aware that all articles and product information presented on BenchChem are intended solely for informational purposes. The products available for purchase on BenchChem are specifically designed for in-vitro studies, which are conducted outside of living organisms. In-vitro studies, derived from the Latin term "in glass," involve experiments performed in controlled laboratory settings using cells or tissues. It is important to note that these products are not categorized as medicines or drugs, and they have not received approval from the FDA for the prevention, treatment, or cure of any medical condition, ailment, or disease. We must emphasize that any form of bodily introduction of these products into humans or animals is strictly prohibited by law. It is essential to adhere to these guidelines to ensure compliance with legal and ethical standards in research and experimentation.