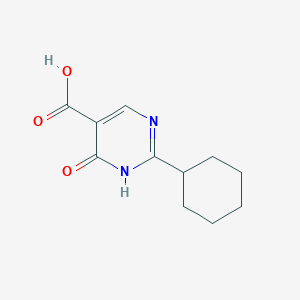
2-Cyclohexyl-6-oxo-1,6-dihydropyrimidine-5-carboxylic acid
- Click on QUICK INQUIRY to receive a quote from our team of experts.
- With the quality product at a COMPETITIVE price, you can focus more on your research.
Overview
Description
2-Cyclohexyl-6-oxo-1,6-dihydropyrimidine-5-carboxylic acid is a compound belonging to the class of dihydropyrimidine derivatives. These compounds are known for their diverse biological activities and have been extensively studied for their potential therapeutic applications. The structure of this compound includes a cyclohexyl group attached to a dihydropyrimidine ring, which is further substituted with a carboxylic acid group.
Preparation Methods
Synthetic Routes and Reaction Conditions
The synthesis of 2-Cyclohexyl-6-oxo-1,6-dihydropyrimidine-5-carboxylic acid typically involves the cyclization of appropriate precursors under specific reaction conditions. One common method involves the reaction of cyclohexanone with urea and ethyl acetoacetate in the presence of a catalyst such as acetic acid. The reaction mixture is heated under reflux conditions to facilitate the cyclization process, leading to the formation of the desired dihydropyrimidine derivative .
Industrial Production Methods
Industrial production methods for this compound may involve similar synthetic routes but are optimized for large-scale production. This includes the use of continuous flow reactors, automated systems, and efficient purification techniques to ensure high yield and purity of the final product .
Chemical Reactions Analysis
Types of Reactions
2-Cyclohexyl-6-oxo-1,6-dihydropyrimidine-5-carboxylic acid undergoes various chemical reactions, including:
Oxidation: The compound can be oxidized to form corresponding oxo derivatives.
Reduction: Reduction reactions can convert the oxo group to a hydroxyl group.
Substitution: The carboxylic acid group can undergo substitution reactions to form esters or amides.
Common Reagents and Conditions
Oxidation: Common oxidizing agents include potassium permanganate (KMnO4) and chromium trioxide (CrO3).
Reduction: Reducing agents such as sodium borohydride (NaBH4) or lithium aluminum hydride (LiAlH4) are used.
Major Products
Oxidation: Formation of this compound derivatives with additional oxo groups.
Reduction: Formation of 2-Cyclohexyl-6-hydroxy-1,6-dihydropyrimidine-5-carboxylic acid.
Substitution: Formation of esters or amides of this compound.
Scientific Research Applications
Chemistry: Used as a building block in the synthesis of more complex molecules.
Biology: Investigated for its potential as an enzyme inhibitor, particularly xanthine oxidase inhibitors.
Medicine: Explored for its therapeutic potential in treating hyperuricemia and related conditions.
Industry: Utilized in the development of new materials and chemical processes.
Mechanism of Action
The mechanism of action of 2-Cyclohexyl-6-oxo-1,6-dihydropyrimidine-5-carboxylic acid involves its interaction with specific molecular targets. For instance, as a xanthine oxidase inhibitor, it binds to the active site of the enzyme, preventing the oxidation of hypoxanthine to xanthine and subsequently to uric acid. This inhibition reduces the production of uric acid, making it useful in the treatment of hyperuricemia .
Comparison with Similar Compounds
Similar Compounds
2-Substituted 6-oxo-1,6-dihydropyrimidine-5-carboxylic acids: These compounds share a similar core structure but differ in the substituents attached to the dihydropyrimidine ring.
Ethyl 6-methyl-2-oxo-4-phenyl-1,2,3,4-tetrahydropyrimidine-5-carboxylate: This compound has a similar dihydropyrimidine ring but with different substituents.
Uniqueness
2-Cyclohexyl-6-oxo-1,6-dihydropyrimidine-5-carboxylic acid is unique due to its specific cyclohexyl substitution, which imparts distinct chemical and biological properties. This uniqueness makes it a valuable compound for targeted therapeutic applications and further research .
Properties
Molecular Formula |
C11H14N2O3 |
---|---|
Molecular Weight |
222.24 g/mol |
IUPAC Name |
2-cyclohexyl-6-oxo-1H-pyrimidine-5-carboxylic acid |
InChI |
InChI=1S/C11H14N2O3/c14-10-8(11(15)16)6-12-9(13-10)7-4-2-1-3-5-7/h6-7H,1-5H2,(H,15,16)(H,12,13,14) |
InChI Key |
KRTPMMPSWNLACG-UHFFFAOYSA-N |
Canonical SMILES |
C1CCC(CC1)C2=NC=C(C(=O)N2)C(=O)O |
Origin of Product |
United States |
Disclaimer and Information on In-Vitro Research Products
Please be aware that all articles and product information presented on BenchChem are intended solely for informational purposes. The products available for purchase on BenchChem are specifically designed for in-vitro studies, which are conducted outside of living organisms. In-vitro studies, derived from the Latin term "in glass," involve experiments performed in controlled laboratory settings using cells or tissues. It is important to note that these products are not categorized as medicines or drugs, and they have not received approval from the FDA for the prevention, treatment, or cure of any medical condition, ailment, or disease. We must emphasize that any form of bodily introduction of these products into humans or animals is strictly prohibited by law. It is essential to adhere to these guidelines to ensure compliance with legal and ethical standards in research and experimentation.