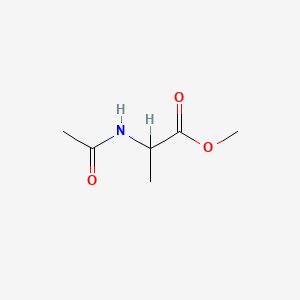
Methyl 2-acetamidopropanoate
Overview
Description
Methyl 2-acetamidopropanoate is an organic compound belonging to the class of alpha amino acid esters. It is characterized by the presence of an acetylamino group attached to the second carbon of a propanoate ester. This compound is widely used in various fields due to its unique chemical properties and reactivity.
Preparation Methods
Synthetic Routes and Reaction Conditions: Methyl 2-acetamidopropanoate can be synthesized through several methods. One common method involves the reaction between glycine ethyl ester hydrochloride and acetic anhydride in the presence of a base such as sodium ethoxide. Another method includes the use of bis(1,5-cyclooctadiene)rhodium(I) tetrafluoroborate as a catalyst in dichloromethane under hydrogen pressure .
Industrial Production Methods: Industrial production of this compound typically involves large-scale synthesis using optimized reaction conditions to ensure high yield and purity. The process often includes the use of automated reactors and stringent quality control measures to maintain consistency.
Chemical Reactions Analysis
Types of Reactions: Methyl 2-acetamidopropanoate undergoes various chemical reactions, including:
Oxidation: The compound can be oxidized to form corresponding carboxylic acids.
Reduction: Reduction reactions can convert the ester group to an alcohol.
Substitution: Nucleophilic substitution reactions can occur at the ester or amide groups.
Common Reagents and Conditions:
Oxidation: Common oxidizing agents include potassium permanganate and chromium trioxide.
Reduction: Sodium borohydride and lithium aluminum hydride are frequently used reducing agents.
Substitution: Reagents such as sodium hydroxide or hydrochloric acid can facilitate substitution reactions.
Major Products Formed:
Oxidation: Carboxylic acids.
Reduction: Alcohols.
Substitution: Various substituted derivatives depending on the nucleophile used.
Scientific Research Applications
Methyl 2-acetamidopropanoate has numerous applications in scientific research:
Chemistry: It serves as a building block for synthesizing peptides and other complex molecules.
Biology: The compound is used in the study of enzyme mechanisms and protein synthesis.
Medicine: It is involved in the development of pharmaceuticals and drug delivery systems.
Industry: The compound is used in the production of specialty chemicals and as an intermediate in various chemical processes.
Mechanism of Action
The mechanism of action of methyl 2-acetamidopropanoate involves its interaction with specific molecular targets. The acetylamino group can participate in hydrogen bonding and other interactions with enzymes and receptors, influencing their activity. The ester group can undergo hydrolysis, releasing the active amino acid derivative, which can then participate in various biochemical pathways .
Comparison with Similar Compounds
- Methyl 2-amino-2-methylpropanoate
- Ethyl 2-acetamidopropanoate
- Methyl 2-acetamidobutanoate
Comparison: Methyl 2-acetamidopropanoate is unique due to its specific ester and amide functional groups, which confer distinct reactivity and stability. Compared to similar compounds, it offers a balance of hydrophilicity and lipophilicity, making it versatile for various applications .
Properties
IUPAC Name |
methyl 2-acetamidopropanoate | |
---|---|---|
Source | PubChem | |
URL | https://pubchem.ncbi.nlm.nih.gov | |
Description | Data deposited in or computed by PubChem | |
InChI |
InChI=1S/C6H11NO3/c1-4(6(9)10-3)7-5(2)8/h4H,1-3H3,(H,7,8) | |
Source | PubChem | |
URL | https://pubchem.ncbi.nlm.nih.gov | |
Description | Data deposited in or computed by PubChem | |
InChI Key |
FQGVVDYNRHNTCK-UHFFFAOYSA-N | |
Source | PubChem | |
URL | https://pubchem.ncbi.nlm.nih.gov | |
Description | Data deposited in or computed by PubChem | |
Canonical SMILES |
CC(C(=O)OC)NC(=O)C | |
Source | PubChem | |
URL | https://pubchem.ncbi.nlm.nih.gov | |
Description | Data deposited in or computed by PubChem | |
Molecular Formula |
C6H11NO3 | |
Source | PubChem | |
URL | https://pubchem.ncbi.nlm.nih.gov | |
Description | Data deposited in or computed by PubChem | |
DSSTOX Substance ID |
DTXSID60949461 | |
Record name | N-(1-Methoxy-1-oxopropan-2-yl)ethanimidic acid | |
Source | EPA DSSTox | |
URL | https://comptox.epa.gov/dashboard/DTXSID60949461 | |
Description | DSSTox provides a high quality public chemistry resource for supporting improved predictive toxicology. | |
Molecular Weight |
145.16 g/mol | |
Source | PubChem | |
URL | https://pubchem.ncbi.nlm.nih.gov | |
Description | Data deposited in or computed by PubChem | |
CAS No. |
26629-33-4 | |
Record name | N-(1-Methoxy-1-oxopropan-2-yl)ethanimidic acid | |
Source | EPA DSSTox | |
URL | https://comptox.epa.gov/dashboard/DTXSID60949461 | |
Description | DSSTox provides a high quality public chemistry resource for supporting improved predictive toxicology. | |
Synthesis routes and methods I
Procedure details
Synthesis routes and methods II
Procedure details
Synthesis routes and methods III
Procedure details
Retrosynthesis Analysis
AI-Powered Synthesis Planning: Our tool employs the Template_relevance Pistachio, Template_relevance Bkms_metabolic, Template_relevance Pistachio_ringbreaker, Template_relevance Reaxys, Template_relevance Reaxys_biocatalysis model, leveraging a vast database of chemical reactions to predict feasible synthetic routes.
One-Step Synthesis Focus: Specifically designed for one-step synthesis, it provides concise and direct routes for your target compounds, streamlining the synthesis process.
Accurate Predictions: Utilizing the extensive PISTACHIO, BKMS_METABOLIC, PISTACHIO_RINGBREAKER, REAXYS, REAXYS_BIOCATALYSIS database, our tool offers high-accuracy predictions, reflecting the latest in chemical research and data.
Strategy Settings
Precursor scoring | Relevance Heuristic |
---|---|
Min. plausibility | 0.01 |
Model | Template_relevance |
Template Set | Pistachio/Bkms_metabolic/Pistachio_ringbreaker/Reaxys/Reaxys_biocatalysis |
Top-N result to add to graph | 6 |
Feasible Synthetic Routes
Disclaimer and Information on In-Vitro Research Products
Please be aware that all articles and product information presented on BenchChem are intended solely for informational purposes. The products available for purchase on BenchChem are specifically designed for in-vitro studies, which are conducted outside of living organisms. In-vitro studies, derived from the Latin term "in glass," involve experiments performed in controlled laboratory settings using cells or tissues. It is important to note that these products are not categorized as medicines or drugs, and they have not received approval from the FDA for the prevention, treatment, or cure of any medical condition, ailment, or disease. We must emphasize that any form of bodily introduction of these products into humans or animals is strictly prohibited by law. It is essential to adhere to these guidelines to ensure compliance with legal and ethical standards in research and experimentation.