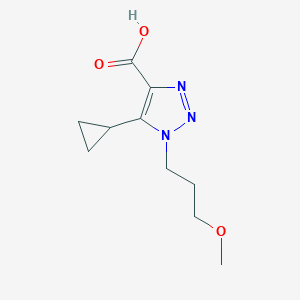
5-Cyclopropyl-1-(3-methoxypropyl)-1h-1,2,3-triazole-4-carboxylic acid
- Click on QUICK INQUIRY to receive a quote from our team of experts.
- With the quality product at a COMPETITIVE price, you can focus more on your research.
Overview
Description
5-Cyclopropyl-1-(3-methoxypropyl)-1h-1,2,3-triazole-4-carboxylic acid is a chemical compound that belongs to the class of triazole derivatives Triazoles are known for their diverse biological activities and are widely used in medicinal chemistry
Preparation Methods
Synthetic Routes and Reaction Conditions
The synthesis of 5-Cyclopropyl-1-(3-methoxypropyl)-1h-1,2,3-triazole-4-carboxylic acid typically involves the following steps:
Formation of the Triazole Ring: The triazole ring can be formed through a cycloaddition reaction between an azide and an alkyne
Introduction of the Cyclopropyl Group: The cyclopropyl group can be introduced through a cyclopropanation reaction, which involves the addition of a carbene to an alkene.
Attachment of the Methoxypropyl Group: The methoxypropyl group can be attached through an alkylation reaction, where a suitable alkylating agent reacts with the triazole ring.
Carboxylation: The carboxylic acid group can be introduced through a carboxylation reaction, where a suitable carboxylating agent reacts with the triazole ring.
Industrial Production Methods
Industrial production of this compound may involve optimizing the above synthetic routes to achieve higher yields and purity. This can include the use of advanced catalysts, optimized reaction conditions, and purification techniques such as chromatography and crystallization.
Chemical Reactions Analysis
Types of Reactions
5-Cyclopropyl-1-(3-methoxypropyl)-1h-1,2,3-triazole-4-carboxylic acid can undergo various types of chemical reactions, including:
Oxidation: The compound can be oxidized to form various oxidation products, depending on the reagents and conditions used.
Reduction: Reduction reactions can convert the compound into different reduced forms.
Substitution: The compound can undergo substitution reactions, where one functional group is replaced by another.
Common Reagents and Conditions
Oxidation: Common oxidizing agents include potassium permanganate, chromium trioxide, and hydrogen peroxide.
Reduction: Common reducing agents include lithium aluminum hydride, sodium borohydride, and hydrogen gas with a suitable catalyst.
Substitution: Common reagents for substitution reactions include halogenating agents, alkylating agents, and nucleophiles.
Major Products
The major products formed from these reactions depend on the specific reagents and conditions used. For example, oxidation may yield carboxylic acids, ketones, or aldehydes, while reduction may yield alcohols or amines.
Scientific Research Applications
5-Cyclopropyl-1-(3-methoxypropyl)-1h-1,2,3-triazole-4-carboxylic acid has several scientific research applications, including:
Medicinal Chemistry: The compound is studied for its potential as a pharmaceutical agent due to its triazole ring, which is known for its biological activity.
Biological Research: The compound is used in biological research to study its effects on various biological systems and pathways.
Industrial Applications: The compound may be used in the development of new materials, catalysts, and other industrial applications.
Mechanism of Action
The mechanism of action of 5-Cyclopropyl-1-(3-methoxypropyl)-1h-1,2,3-triazole-4-carboxylic acid involves its interaction with specific molecular targets and pathways. The triazole ring can interact with enzymes, receptors, and other proteins, leading to various biological effects. The exact mechanism of action depends on the specific application and target.
Comparison with Similar Compounds
Similar Compounds
1,2,3-Triazole Derivatives: Other compounds with the 1,2,3-triazole ring, such as 1-phenyl-1h-1,2,3-triazole-4-carboxylic acid.
Cyclopropyl Derivatives: Compounds with the cyclopropyl group, such as cyclopropylamine.
Methoxypropyl Derivatives: Compounds with the methoxypropyl group, such as 3-methoxypropylamine.
Uniqueness
5-Cyclopropyl-1-(3-methoxypropyl)-1h-1,2,3-triazole-4-carboxylic acid is unique due to its combination of the cyclopropyl, methoxypropyl, and triazole groups. This unique structure gives it distinct chemical and biological properties, making it a valuable compound for research and industrial applications.
Properties
Molecular Formula |
C10H15N3O3 |
---|---|
Molecular Weight |
225.24 g/mol |
IUPAC Name |
5-cyclopropyl-1-(3-methoxypropyl)triazole-4-carboxylic acid |
InChI |
InChI=1S/C10H15N3O3/c1-16-6-2-5-13-9(7-3-4-7)8(10(14)15)11-12-13/h7H,2-6H2,1H3,(H,14,15) |
InChI Key |
TWXXGMKNUUYAOG-UHFFFAOYSA-N |
Canonical SMILES |
COCCCN1C(=C(N=N1)C(=O)O)C2CC2 |
Origin of Product |
United States |
Disclaimer and Information on In-Vitro Research Products
Please be aware that all articles and product information presented on BenchChem are intended solely for informational purposes. The products available for purchase on BenchChem are specifically designed for in-vitro studies, which are conducted outside of living organisms. In-vitro studies, derived from the Latin term "in glass," involve experiments performed in controlled laboratory settings using cells or tissues. It is important to note that these products are not categorized as medicines or drugs, and they have not received approval from the FDA for the prevention, treatment, or cure of any medical condition, ailment, or disease. We must emphasize that any form of bodily introduction of these products into humans or animals is strictly prohibited by law. It is essential to adhere to these guidelines to ensure compliance with legal and ethical standards in research and experimentation.