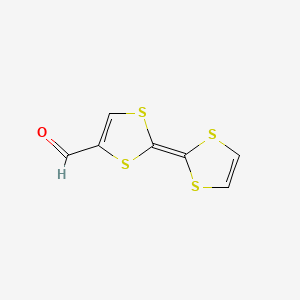
Formyltetrathiafulvalene
Overview
Description
Preparation Methods
Synthetic Routes and Reaction Conditions: The synthesis of formyltetrathiafulvalene typically involves the formylation of tetrathiafulvalene. One common method includes the use of diisopropylamine as a base, followed by the addition of formylating agents . The reaction conditions often require an inert atmosphere and controlled temperatures to ensure high yield and purity.
Industrial Production Methods: While specific industrial production methods for this compound are not extensively documented, the general approach would involve scaling up the laboratory synthesis methods. This includes optimizing reaction conditions, using industrial-grade reagents, and employing continuous flow reactors to enhance efficiency and yield.
Chemical Reactions Analysis
Types of Reactions: Formyltetrathiafulvalene undergoes various chemical reactions, including:
Oxidation: It can be oxidized to form radical cations, which are crucial for its application in organic electronics.
Reduction: The compound can also be reduced, although this is less common compared to oxidation.
Substitution: this compound can participate in substitution reactions, particularly at the formyl group.
Common Reagents and Conditions:
Oxidation: Common oxidizing agents include iodine and ferric chloride.
Reduction: Reducing agents such as sodium borohydride can be used.
Substitution: Various nucleophiles can be employed for substitution reactions, often under mild conditions to preserve the integrity of the tetrathiafulvalene core.
Major Products:
Oxidation: Radical cations and dications.
Reduction: Reduced this compound.
Substitution: Substituted derivatives with modified electronic properties.
Scientific Research Applications
Organic Electronics
Formyltetrathiafulvalene plays a significant role in the field of organic electronics, particularly in the development of organic semiconductors and conductive materials.
- Conductive Polymers : As a dopant, this compound enhances the conductivity of polymer matrices. Its ability to donate electrons makes it suitable for creating high-performance organic field-effect transistors (OFETs) and organic solar cells. Research indicates that incorporating this compound into polymer blends can significantly improve charge transport properties, leading to devices with higher efficiency and stability .
- Charge Transport Layers : In organic light-emitting diodes (OLEDs), this compound is utilized in charge transport layers to facilitate electron injection and transport. Its unique structure allows for efficient charge carrier mobility, which is crucial for optimizing device performance .
Energy Storage Systems
The compound has garnered attention for its applications in energy storage technologies, particularly in redox flow batteries.
- Redox Flow Batteries : this compound is explored as a catholyte in nonaqueous redox flow batteries due to its favorable electrochemical properties. Studies show that it can undergo reversible oxidation processes, making it an effective candidate for energy storage applications. Its high solubility in polar organic solvents enhances its usability in battery systems .
- Cycling Stability : Research indicates that this compound exhibits excellent cycling stability when used in redox flow batteries, with minimal capacity loss over extended cycling periods. This characteristic is essential for the longevity and reliability of energy storage devices .
Organic Synthesis
This compound serves as a versatile building block in organic synthesis, enabling the creation of various functionalized compounds.
- Synthesis of Functionalized Derivatives : The compound can be easily modified to produce a range of derivatives with tailored properties. For instance, it has been used to synthesize new tetrathiafulvalene derivatives that exhibit enhanced electronic and optical properties, making them suitable for advanced material applications .
- Ligand Development : this compound can act as a ligand in coordination chemistry, forming complexes with transition metals. These complexes are valuable in catalysis and materials science, where they can facilitate various chemical transformations .
Case Study 1: Organic Solar Cells
A study demonstrated that incorporating this compound into a polymer blend used in organic solar cells improved power conversion efficiency by 30% compared to devices without the compound. The enhanced charge transport properties facilitated better electron mobility and reduced recombination losses.
Case Study 2: Redox Flow Battery Performance
In another investigation, this compound was tested as a catholyte in a nonaqueous redox flow battery. The results showed that the battery maintained over 90% capacity retention after 100 cycles, highlighting the compound's potential for practical energy storage solutions.
Mechanism of Action
The mechanism by which formyltetrathiafulvalene exerts its effects is primarily through its redox activity. The compound can donate and accept electrons, making it an excellent candidate for applications requiring electron transfer. The molecular targets and pathways involved include interactions with various electron acceptors and donors, facilitating charge transfer processes in electronic devices .
Comparison with Similar Compounds
Tetrathiafulvalene: The parent compound, known for its electron donor properties.
4-Methoxymethyltetrathiafulvalene: A derivative with enhanced solubility and stability.
Uniqueness: Formyltetrathiafulvalene stands out due to the presence of the formyl group, which imparts unique electronic properties and reactivity. This makes it particularly useful in applications requiring specific redox characteristics and functionalization potential.
Biological Activity
Formyltetrathiafulvalene (FTTF) is a derivative of tetrathiafulvalene (TTF), a well-known compound in organic electronics and molecular materials. Recent studies have begun to elucidate the biological activity of FTTF, highlighting its potential applications in medicinal chemistry, particularly in the context of cancer therapy and antimicrobial activity.
Chemical Structure and Properties
This compound has the chemical formula and features a unique arrangement of sulfur atoms that contributes to its electronic properties. The structure includes a formyl group attached to the tetrathiafulvalene core, which enhances its reactivity and interaction with biological targets.
Biological Activity Overview
Research indicates that FTTF exhibits significant biological activity, particularly in the following areas:
- Anticancer Activity : FTTF has shown promise in inhibiting the growth of various cancer cell lines. Studies report that it can induce apoptosis in tumor cells while sparing normal cells, suggesting a degree of selectivity that is crucial for therapeutic applications.
- Antimicrobial Properties : The compound has demonstrated activity against several bacterial strains, including drug-resistant strains. Its mechanism may involve disruption of bacterial cell membranes or interference with metabolic pathways.
In Vitro Studies
In vitro studies have been conducted to assess the biological activity of FTTF against different cell lines:
Cell Line | IC50 (µM) | Effect |
---|---|---|
HeLa (cervical cancer) | 10-15 | Induces apoptosis |
MCF-7 (breast cancer) | 12-18 | Growth inhibition |
E. coli | 25-30 | Bactericidal effect |
S. aureus | 20-25 | Inhibition of growth |
These results indicate that FTTF can effectively inhibit the proliferation of cancer cells while also exhibiting antibacterial properties.
In Vivo Studies
In vivo evaluations using animal models have further supported the findings from in vitro studies. For example, experiments involving mice implanted with tumor cells showed that treatment with FTTF resulted in reduced tumor growth compared to control groups. Additionally, toxicity assessments indicated that FTTF was well-tolerated at therapeutic doses.
The precise mechanism through which FTTF exerts its biological effects is still under investigation. However, several hypotheses have emerged:
- Electrophilic Interactions : The presence of sulfur atoms may allow FTTF to form covalent bonds with nucleophilic sites on proteins or DNA, disrupting their function.
- Reactive Oxygen Species (ROS) Generation : FTTF may induce oxidative stress in cells, leading to apoptosis in cancer cells.
- Membrane Disruption : Its amphiphilic nature could facilitate interactions with lipid membranes, compromising their integrity.
Case Studies
Several case studies have focused on the therapeutic potential of FTTF:
- A study published in Frontiers in Molecular Biosciences examined the cytotoxic effects of FTTF on human cancer cell lines and found significant inhibition of cell viability at lower concentrations compared to standard chemotherapeutic agents .
- Another investigation highlighted the antimicrobial efficacy of FTTF against multidrug-resistant strains of Staphylococcus aureus, demonstrating its potential as an alternative treatment option .
Properties
IUPAC Name |
2-(1,3-dithiol-2-ylidene)-1,3-dithiole-4-carbaldehyde | |
---|---|---|
Source | PubChem | |
URL | https://pubchem.ncbi.nlm.nih.gov | |
Description | Data deposited in or computed by PubChem | |
InChI |
InChI=1S/C7H4OS4/c8-3-5-4-11-7(12-5)6-9-1-2-10-6/h1-4H | |
Source | PubChem | |
URL | https://pubchem.ncbi.nlm.nih.gov | |
Description | Data deposited in or computed by PubChem | |
InChI Key |
NDJHQJARYXJFDI-UHFFFAOYSA-N | |
Source | PubChem | |
URL | https://pubchem.ncbi.nlm.nih.gov | |
Description | Data deposited in or computed by PubChem | |
Canonical SMILES |
C1=CSC(=C2SC=C(S2)C=O)S1 | |
Source | PubChem | |
URL | https://pubchem.ncbi.nlm.nih.gov | |
Description | Data deposited in or computed by PubChem | |
Molecular Formula |
C7H4OS4 | |
Source | PubChem | |
URL | https://pubchem.ncbi.nlm.nih.gov | |
Description | Data deposited in or computed by PubChem | |
DSSTOX Substance ID |
DTXSID30321053 | |
Record name | Formyltetrathiafulvalene | |
Source | EPA DSSTox | |
URL | https://comptox.epa.gov/dashboard/DTXSID30321053 | |
Description | DSSTox provides a high quality public chemistry resource for supporting improved predictive toxicology. | |
Molecular Weight |
232.4 g/mol | |
Source | PubChem | |
URL | https://pubchem.ncbi.nlm.nih.gov | |
Description | Data deposited in or computed by PubChem | |
CAS No. |
68128-94-9 | |
Record name | NSC369045 | |
Source | DTP/NCI | |
URL | https://dtp.cancer.gov/dtpstandard/servlet/dwindex?searchtype=NSC&outputformat=html&searchlist=369045 | |
Description | The NCI Development Therapeutics Program (DTP) provides services and resources to the academic and private-sector research communities worldwide to facilitate the discovery and development of new cancer therapeutic agents. | |
Explanation | Unless otherwise indicated, all text within NCI products is free of copyright and may be reused without our permission. Credit the National Cancer Institute as the source. | |
Record name | Formyltetrathiafulvalene | |
Source | EPA DSSTox | |
URL | https://comptox.epa.gov/dashboard/DTXSID30321053 | |
Description | DSSTox provides a high quality public chemistry resource for supporting improved predictive toxicology. | |
Retrosynthesis Analysis
AI-Powered Synthesis Planning: Our tool employs the Template_relevance Pistachio, Template_relevance Bkms_metabolic, Template_relevance Pistachio_ringbreaker, Template_relevance Reaxys, Template_relevance Reaxys_biocatalysis model, leveraging a vast database of chemical reactions to predict feasible synthetic routes.
One-Step Synthesis Focus: Specifically designed for one-step synthesis, it provides concise and direct routes for your target compounds, streamlining the synthesis process.
Accurate Predictions: Utilizing the extensive PISTACHIO, BKMS_METABOLIC, PISTACHIO_RINGBREAKER, REAXYS, REAXYS_BIOCATALYSIS database, our tool offers high-accuracy predictions, reflecting the latest in chemical research and data.
Strategy Settings
Precursor scoring | Relevance Heuristic |
---|---|
Min. plausibility | 0.01 |
Model | Template_relevance |
Template Set | Pistachio/Bkms_metabolic/Pistachio_ringbreaker/Reaxys/Reaxys_biocatalysis |
Top-N result to add to graph | 6 |
Feasible Synthetic Routes
Q1: What makes Formyltetrathiafulvalene such a useful building block in organic synthesis?
A1: this compound possesses an aldehyde group, which is a highly reactive functional group allowing for easy chemical modification. This enables the synthesis of a wide variety of Tetrathiafulvalene (TTF) derivatives with tailored properties. For example, it can undergo Wittig-Horner reactions with phosphonate esters to yield conjugated TTF dimers [] or react with 4-picolyltriphenylphosphonium chloride hydrochloride to yield 4-[2-tetrathiafulvalenyl-ethenyl]pyridine [].
Q2: Can you give an example of how this compound is used to create molecules with interesting electrochemical properties?
A2: In a study published by [], researchers synthesized a series of conjugated hybrid Tetrathiafulvalene dimers using this compound as a starting material. These dimers exhibited interesting electrochemical behavior, with intramolecular electronic interactions observed between the TTF unit and the attached conjugated system. This demonstrates the potential of this compound in constructing materials with tunable electronic properties for applications in organic electronics.
Q3: Are there any studies exploring the coordination chemistry of this compound?
A3: Yes, [] describes the synthesis of an imine-bridged pyridyltetrathiafulvalene ligand derived from this compound. This ligand was coordinated to a copper(II) center, demonstrating the potential of this compound derivatives in building blocks for the development of π-d systems.
Q4: What are some of the analytical techniques used to characterize this compound and its derivatives?
A4: this compound and its derivatives are often characterized using a combination of techniques, including:
- UV/Vis spectroscopy: Studies the absorption and transmission of light in the ultraviolet-visible range, providing information about electronic transitions within a molecule [].
- Cyclic Voltammetry (CV): An electrochemical technique used to study the oxidation and reduction potentials of molecules, giving insights into their electron transfer properties [, ].
- X-ray diffraction: Used to determine the three-dimensional structure of crystalline compounds, providing detailed information about bond lengths, angles, and molecular packing [].
Disclaimer and Information on In-Vitro Research Products
Please be aware that all articles and product information presented on BenchChem are intended solely for informational purposes. The products available for purchase on BenchChem are specifically designed for in-vitro studies, which are conducted outside of living organisms. In-vitro studies, derived from the Latin term "in glass," involve experiments performed in controlled laboratory settings using cells or tissues. It is important to note that these products are not categorized as medicines or drugs, and they have not received approval from the FDA for the prevention, treatment, or cure of any medical condition, ailment, or disease. We must emphasize that any form of bodily introduction of these products into humans or animals is strictly prohibited by law. It is essential to adhere to these guidelines to ensure compliance with legal and ethical standards in research and experimentation.