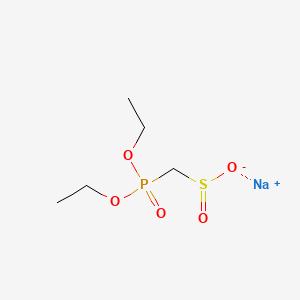
Sodium(diethoxyphosphoryl)methanesulfinate
- Click on QUICK INQUIRY to receive a quote from our team of experts.
- With the quality product at a COMPETITIVE price, you can focus more on your research.
Overview
Description
Sodium (diethoxyphosphoryl)methanesulfinate is a chemical compound with a unique structure that includes both sulfonate and phosphoryl groups
Preparation Methods
Synthetic Routes and Reaction Conditions
The synthesis of sodium (diethoxyphosphoryl)methanesulfinate typically involves the reaction of methanesulfinic acid with diethoxyphosphoryl chloride in the presence of a base such as sodium hydroxide. The reaction is carried out under controlled conditions to ensure the formation of the desired product. The general reaction scheme can be represented as follows:
CH3SO2H+(C2H5O)2PCl+NaOH→CH3SO2P(O)(OC2H5)2Na+H2O+NaCl
Industrial Production Methods
Industrial production of sodium (diethoxyphosphoryl)methanesulfinate may involve similar synthetic routes but on a larger scale. The process would include steps such as purification and crystallization to obtain the compound in high purity. The use of continuous flow reactors and automated systems can enhance the efficiency and yield of the production process.
Chemical Reactions Analysis
Types of Reactions
Sodium (diethoxyphosphoryl)methanesulfinate can undergo various types of chemical reactions, including:
Oxidation: The compound can be oxidized to form sulfonates and phosphonates.
Reduction: It can be reduced to form sulfides and phosphines.
Substitution: The compound can participate in nucleophilic substitution reactions, where the sulfonate or phosphoryl group is replaced by other nucleophiles.
Common Reagents and Conditions
Common reagents used in these reactions include oxidizing agents like hydrogen peroxide, reducing agents like lithium aluminum hydride, and nucleophiles such as amines and alcohols. The reactions are typically carried out under mild to moderate conditions to prevent decomposition of the compound.
Major Products
The major products formed from these reactions include sulfonates, phosphonates, sulfides, and phosphines, depending on the specific reaction conditions and reagents used.
Scientific Research Applications
Sodium (diethoxyphosphoryl)methanesulfinate has several scientific research applications, including:
Organic Synthesis: It is used as a reagent in the synthesis of various organosulfur and organophosphorus compounds.
Biology: The compound can be used in biochemical studies to investigate the role of sulfonate and phosphoryl groups in biological systems.
Industry: The compound is used in the production of specialty chemicals and materials, including polymers and surfactants.
Mechanism of Action
The mechanism of action of sodium (diethoxyphosphoryl)methanesulfinate involves the interaction of its sulfonate and phosphoryl groups with various molecular targets. The sulfonate group can act as a nucleophile, participating in substitution reactions, while the phosphoryl group can form strong bonds with metal ions and other electrophiles. These interactions can lead to the formation of stable complexes and the modulation of biochemical pathways.
Comparison with Similar Compounds
Similar Compounds
Similar compounds to sodium (diethoxyphosphoryl)methanesulfinate include:
- Sodium methanesulfinate
- Sodium ethylphosphonate
- Sodium diethylphosphinate
Uniqueness
Sodium (diethoxyphosphoryl)methanesulfinate is unique due to the presence of both sulfonate and phosphoryl groups in its structure. This dual functionality allows it to participate in a wider range of chemical reactions compared to compounds with only one of these groups. Additionally, the presence of ethoxy groups enhances its solubility and reactivity in organic solvents.
Properties
Molecular Formula |
C5H12NaO5PS |
---|---|
Molecular Weight |
238.18 g/mol |
IUPAC Name |
sodium;diethoxyphosphorylmethanesulfinate |
InChI |
InChI=1S/C5H13O5PS.Na/c1-3-9-11(6,10-4-2)5-12(7)8;/h3-5H2,1-2H3,(H,7,8);/q;+1/p-1 |
InChI Key |
IUNXKJCPIBLYEW-UHFFFAOYSA-M |
Canonical SMILES |
CCOP(=O)(CS(=O)[O-])OCC.[Na+] |
Origin of Product |
United States |
Disclaimer and Information on In-Vitro Research Products
Please be aware that all articles and product information presented on BenchChem are intended solely for informational purposes. The products available for purchase on BenchChem are specifically designed for in-vitro studies, which are conducted outside of living organisms. In-vitro studies, derived from the Latin term "in glass," involve experiments performed in controlled laboratory settings using cells or tissues. It is important to note that these products are not categorized as medicines or drugs, and they have not received approval from the FDA for the prevention, treatment, or cure of any medical condition, ailment, or disease. We must emphasize that any form of bodily introduction of these products into humans or animals is strictly prohibited by law. It is essential to adhere to these guidelines to ensure compliance with legal and ethical standards in research and experimentation.