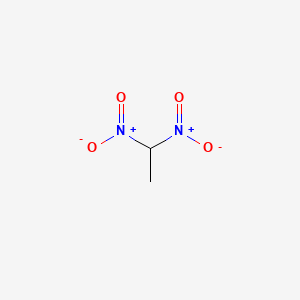
1,1-Dinitroethane
Overview
Description
1,1-Dinitroethane (DNE, C₂H₄N₂O₄) is a nitroalkane with the CAS Registry Number 600-40-6. It has a molecular mass of 120.06 g/mol and exhibits a density of 1.355 g/cm³ at 20°C . Key physical properties include a melting point of 121.2–121.6°C and a boiling point of 185.5°C . Chemically, it is characterized by two nitro groups (-NO₂) attached to the same carbon atom, contributing to its high polarity and reactivity.
DNE is utilized in polymer solutions (e.g., polyethylene-co-vinyl acetate) for biomedical applications and as a precursor in the synthesis of nitrohydrocarbons via addition reactions with nitroalkenes . It is also a degradation product of the plasticizer bis(2,2-dinitropropyl) acetal/formal (BDNPA/F), highlighting its role in material stability studies . Notably, DNE has been reported as a constituent in celery volatiles, though this finding requires further validation .
Preparation Methods
Electrochemical Nitration Method
Overview:
One advanced and efficient method for synthesizing 1,1-dinitroethane involves electrochemical nitration, which uses an electrochemical cell to oxidize chemical mediators that facilitate the nitration of 1-nitroethane.
- A platinum-coated titanium anode and a cathode are used in a three-compartment batch electrolysis cell.
- The anode solution contains potassium ferrocyanide, which dissociates into potassium ions and ferrocyanide ions.
- At the anode, ferrocyanide ions are oxidized to ferricyanide ions, which then react with 1-nitroethane and potassium nitrite to form this compound.
- The reaction is conducted at a low cell potential (~0.6 V) and under continuous stirring with temperature control (around 0°C).
- The this compound product precipitates as a potassium salt, which can be isolated by cooling and filtration.
- The process achieves a yield of approximately 69% with a current efficiency near 91%.
- The chemical mediator (ferrocyanide) is reusable, reducing waste and cost.
- Lower cell potentials reduce energy consumption compared to direct oxidation methods.
- The method minimizes undesirable by-products and avoids the use of additional chemical oxidizers.
Parameter | Value/Condition |
---|---|
Anode material | Platinum-coated titanium |
Voltage applied | ~0.6 V |
Electrolyte mediator | Potassium ferrocyanide |
Temperature | ~0°C (chilled container) |
Yield of this compound | ~69% |
Current efficiency | ~91% |
Product isolation | Precipitation as potassium salt |
This method also allows subsequent reactions, such as formaldehyde condensation, to produce derivatives with high efficiency.
Chemical Oxidative Nitration Using Nitrite Salts and Oxidizing Agents
Overview:
A classical and widely reported method involves the reaction of nitroethane or its metal salts with nitrite ions in the presence of an oxidizing agent and catalytic amounts of alkali metal ferricyanide.
- Nitroethane is first converted to its metal salt (commonly sodium salt) by reaction with alkali metal hydroxides or carbonates.
- The metal salt solution is then treated with sodium nitrite and an oxidizing agent such as sodium persulfate or potassium persulfate.
- Alkali metal ferricyanide acts as a catalyst to facilitate the oxidation of ferrocyanide to ferricyanide, which promotes the nitration reaction.
- The reaction is typically carried out in aqueous solution at temperatures ranging from 0°C to 100°C under atmospheric pressure.
- Solvents like water or inert alcohols (methanol, ethanol) are used depending on solubility and reactivity considerations.
- After reaction completion, the mixture is acidified to liberate this compound, which can be extracted with organic solvents and purified.
Reaction Scheme:
$$
\text{R-CH}2\text{-NO}2 + \text{NO}2^- \xrightarrow[\text{oxidizing agent}]{\text{alkali metal ferricyanide}} \text{R-C(NO2)_2H}
$$
- The molar ratio of nitrite salt to nitro compound ranges from 1:1 to 5:1.
- Ferricyanide catalyst is used in 0.05 to 0.5 moles per mole of nitro compound.
- Oxidizing agent is used in excess (3 to 10 moles per mole of ferricyanide).
- Typical yields for this compound are reported as moderate to good (50-80%).
Parameter | Typical Range/Value |
---|---|
Nitro compound to hydroxide | ~1:1 molar ratio |
Nitro compound to nitrite salt | 1:1 to 1:5 molar ratio |
Ferricyanide catalyst | 0.05 to 0.5 mol/mol nitro compound |
Oxidizing agent (e.g., Na2S2O8) | 3 to 10 mol/mol ferricyanide |
Temperature | 0–100°C (commonly 0–50°C) |
Solvent | Water, methanol, ethanol |
Yield | 50–80% |
- The reaction is exothermic and often requires cooling to control temperature.
- The method is versatile and applicable to cyclic and acyclic nitro compounds for geminal dinitro synthesis.
- The use of ferricyanide as a catalyst improves selectivity and reduces side reactions.
Silver Salt Mediated Nitration (Shechter-Kaplan Reaction)
Overview:
An older but effective method involves the reaction of nitroalkanes with silver nitrate and sodium nitrite under alkaline conditions, followed by acidification and extraction.
- Sodium hydroxide is dissolved in water, and nitroethane along with sodium nitrite is added to form a reaction mixture.
- This mixture is rapidly added to a stirred aqueous solution of silver nitrate at low temperature (0°C to ice bath).
- After stirring, the mixture is treated with additional sodium hydroxide, and silver salts precipitate out.
- The filtrate containing the sodium salt of this compound is acidified to liberate the free compound.
- The product is extracted with ether and purified by distillation or steam distillation.
- Yields reported for this compound via this method are around 54% to 79%, depending on scale and purification method.
- Larger scale reactions may face issues such as foaming and silver metal precipitation, which can be mitigated by isolating the sodium salt before acidification.
Parameter | Value/Condition |
---|---|
Sodium hydroxide | ~0.5 mole per 0.5 mole nitroethane |
Sodium nitrite | ~0.5 mole per 0.5 mole nitroethane |
Silver nitrate | ~0.5 mole per 0.5 mole nitroethane |
Temperature | 0°C to ice bath |
Product isolation | Acidification, ether extraction, steam distillation |
Yield | 54–79% |
- The method is classical and well-documented but involves handling silver salts, which can be costly and generate metal waste.
- The process requires careful control of pH and temperature to optimize yield.
Summary Table of Preparation Methods for this compound
Method | Key Reagents | Temperature Range | Yield (%) | Advantages | Disadvantages |
---|---|---|---|---|---|
Electrochemical Nitration | 1-nitroethane, K ferrocyanide, K nitrite | ~0°C | ~69 | High efficiency, reusable mediator, low waste | Requires specialized electrochemical setup |
Chemical Oxidative Nitration | Nitroethane salt, NaNO2, oxidizing agent (Na2S2O8), ferricyanide catalyst | 0–100°C | 50–80 | Versatile, catalytic, mild conditions | Exothermic, requires oxidizer handling |
Silver Salt Mediated Nitration | Nitroethane, NaOH, NaNO2, AgNO3 | 0°C to ice bath | 54–79 | Established method, good yields | Silver waste, costly reagents, foaming issues |
This comprehensive analysis integrates data from electrochemical patents, classical synthetic procedures, and chemical oxidation methods to provide a detailed understanding of the preparation of this compound. Each method has its own operational considerations, advantages, and limitations, allowing selection based on scale, available equipment, and desired purity.
The electrochemical method stands out for modern applications due to its environmental and cost benefits, while classical chemical methods remain valuable for laboratory-scale synthesis.
Chemical Reactions Analysis
Types of Reactions: 1,1-Dinitroethane undergoes various chemical reactions, including:
Oxidation: It can be oxidized to form nitroacetic acid.
Reduction: Reduction of this compound can yield 1,1-diaminoethane.
Substitution: It can undergo nucleophilic substitution reactions where the nitro groups are replaced by other functional groups.
Common Reagents and Conditions:
Oxidation: Common oxidizing agents include potassium permanganate and chromium trioxide.
Reduction: Reducing agents such as lithium aluminum hydride or hydrogen in the presence of a catalyst are used.
Substitution: Nucleophiles like hydroxide ions or amines can be used under basic conditions.
Major Products Formed:
Oxidation: Nitroacetic acid.
Reduction: 1,1-Diaminoethane.
Substitution: Various substituted ethane derivatives depending on the nucleophile used.
Scientific Research Applications
1,1-Dinitroethane is a geminal dinitro compound, a class of organic compounds featuring two nitro (-NO2) functional groups attached to the same carbon atom . Geminal dinitro compounds are precursors for energetic plasticizers used in the manufacture of explosive materials and propellant compositions for defense and industrial applications . this compound is also an enolizable compound with an acid strength approximately equal to acetic acid .
Synthesis of Geminal Dinitro Compounds
This compound can be synthesized through the oxidative nitration of a nitroparaffin starting material . One method involves the chemical oxidation of 2-nitroethane . In this process, an oxidizing agent is formed by electrochemically oxidizing a chemical mediator, which then reacts with a nitro compound and a nitrite ion source to yield the geminal dinitro compound .
Electrochemical Nitration
Electrochemical nitration is a method used to synthesize geminal dinitro compounds from nitro compounds . This method involves forming an oxidizing agent by electrochemically oxidizing a chemical mediator and reacting a nitro compound with the oxidizing agent and a nitrite ion source to form a geminal dinitro compound .
Electrochemical Cell Setup
The electrochemical cell includes an anode compartment, which may contain a platinum-coated titanium anode . A voltage of approximately 0.6 V is applied continuously between the anode and cathode . For example, potassium ferrocyanide can dissociate into potassium ions and ferrocyanide ions, with the latter being oxidized to ferricyanide ions at the anode . These ferricyanide ions then react with 1-nitroethane and potassium nitrite to produce this compound .
Advantages of Electrochemical Nitration
This method provides a greater yield of this compound and operates at lower cell potentials compared to direct oxidation on an electrode . By continuously reusing a chemical mediator like ferrocyanide, the method reduces the formation of undesirable waste products and eliminates the need for additional chemical oxidizers, making the process more cost-effective .
Production of DNPOH
The synthesized this compound can be reacted with formaldehyde (H2CO) to produce 2,2-dinitro-1-propanol (DNPOH), with a yield of 69% and a current efficiency of 100% . In one experiment, the this compound product was reacted with excess formaldehyde, resulting in a total product yield of DNPOH of 57%, with a current efficiency of 91% .
Applications in Energetic Materials
Geminal dinitro compounds, including this compound, are essential precursors for energetic plasticizers used in manufacturing explosive materials and propellant compositions for defense and industrial applications .
Examples of Energetic Plasticizers
This compound can be used in the synthesis of various energetic materials, including:
- DNPOH (2,2-dinitro-1-propanol)
- BDNPA (bis(dinitropropyl)-acetal)
- BDNPF (bis(2,2-dinitropropyl)-acetal/bis(2,2-dinitropropyl)formal)
Other Synthesized Compounds
The electrochemical nitration reaction can be employed to synthesize compounds such as:
- 1,3,3-trinitroazetidine (TNAZ)
- 2,2-dinitropropane
- 2,2-dinitropropyl methyl ester
- 3,3-dinitrooxetane
- 2,2-dinitro-1,3-diethoxypropane
- 1,1-dinitrocyclohexane
- 1,1-dinitrocyclopentane
- 2,2-dimethyl-5,5-dinitro-1,3-dioxane
- 1,1-dinitrobutane
- 2,2-dinitrobutane
- 2-cyano-2-nitropropane
- 1-nitro-1-(phenylsulfonyl)cyclohexane
Chemical Properties and Reactions
Mechanism of Action
The mechanism of action of 1,1-Dinitroethane involves its interaction with various molecular targets. The nitro groups can undergo redox reactions, leading to the formation of reactive intermediates. These intermediates can interact with cellular components, leading to oxidative stress and potential cellular damage. The pathways involved include the generation of reactive oxygen species and the subsequent activation of stress response pathways.
Comparison with Similar Compounds
Structural and Thermochemical Properties
Table 1: Physical and Thermochemical Properties of Dinitroalkanes
Compound | Molecular Formula | Molecular Mass (g/mol) | ΔfH° (Exp., kJ/mol) | ΔfH° (Model, kJ/mol) | ABS (Model-Exp) |
---|---|---|---|---|---|
1,1-Dinitroethane | C₂H₄N₂O₄ | 120.06 | -87.2 | -122.36 | 35.16 |
1,1-Dinitropropane | C₃H₆N₂O₄ | 134.09 | -109.5 | -142.99 | 33.49 |
1,1-Dinitrobutane | C₄H₈N₂O₄ | 148.11 | -132.1 | -163.62 | 31.52 |
2,2-Dinitropropane | C₃H₆N₂O₄ | 134.09 | -135.0 | -185.72 | 50.72 |
Key Observations :
- Thermochemical Stability : The experimental enthalpy of formation (ΔfH°) for this compound (-87.2 kJ/mol) is less negative than its higher homologs (e.g., -132.1 kJ/mol for 1,1-dinitrobutane), indicating lower stability .
- Structural Effects : 2,2-Dinitropropane exhibits a larger discrepancy between experimental and modeled ΔfH° (ABS = 50.72 kJ/mol) compared to this compound (ABS = 35.16 kJ/mol), suggesting greater complexity in modeling branched nitroalkanes .
Fluorinated Derivatives
Table 2: Fluorinated Analogues of this compound
Key Observations :
- Impact of Fluorination : Introducing fluorine atoms reduces shock sensitivity. For example, 1-fluoro-1,1-dinitroethane (FDNE) and its difluoro derivative (FDNEF) are less sensitive to detonation than DNE .
- Thermal Stability : Fluorinated derivatives exhibit comparable boiling points to DNE but may decompose into stable fragments like the 1,1-dinitroethanide ion (C₂H₃N₂O₄⁻), as observed in aged plasticizers .
Reactivity and Degradation
Table 3: Reactivity and Decomposition Pathways
Compound | pKa (Aqueous) | Degradation Products | Applications |
---|---|---|---|
This compound | ~8.5 | DNE⁻ fragment (C₂H₃N₂O₄⁻) | Polymer plasticizers, synthetic chemistry |
BDNPA/F | N/A | DNE, formaldehyde | Energetic materials |
Key Observations :
- Acidity : DNE has a pKa of ~8.5, making it a moderately strong carbon acid. Its deprotonation forms the stable dinitroethanide ion, which is detected in decomposition studies .
- Degradation : DNE is a key degradation product of BDNPA/F, indicating its role in material aging and instability .
Table 4: Shock Sensitivity Ranking
Compound | Relative Sensitivity (vs DNE) |
---|---|
FEFO | Higher |
DNE | Baseline |
FDNE/FDNEF | Lower |
Key Observations :
- DNE is more shock-sensitive than its fluorinated derivatives but less sensitive than bis(1-fluoro-1,1-dinitroethyl) formal (FEFO) .
Biological Activity
1,1-Dinitroethane (DNE) is a chemical compound with the formula that has garnered interest due to its unique biological activities and potential applications in various fields. This article explores the biological activity of DNE, summarizing key research findings, case studies, and relevant data.
This compound is characterized by two nitro groups attached to an ethane backbone. Its molecular weight is approximately 104.06 g/mol. The presence of nitro groups significantly influences its reactivity and interaction with biological systems, making it a subject of interest in both chemical research and industrial applications.
Biological Activity Overview
The biological activity of this compound has been investigated in various studies, revealing its potential effects on cellular mechanisms and pathways. The compound exhibits significant reactivity due to the presence of both nitro groups, which can participate in redox reactions within biological systems.
Key Findings
- Cellular Mechanisms : DNE has been shown to affect cellular processes, although detailed biological assays are still limited. It is hypothesized that the nitro groups can influence cellular signaling pathways and metabolic processes .
- Toxicity Studies : Research indicates that DNE may exhibit cytotoxic effects at higher concentrations. Studies have demonstrated that exposure to DNE can lead to oxidative stress in cells, which may contribute to its toxicity .
- Reactivity : The compound's reactivity allows it to form complexes with various biological molecules, potentially altering their function. For instance, DNE has been studied for its interactions with proteins and nucleic acids .
Case Study 1: Cytotoxicity Assessment
A study assessed the cytotoxic effects of this compound on human cell lines. Results indicated that at concentrations above 100 µM, DNE significantly reduced cell viability and induced apoptosis. The mechanism was linked to oxidative stress and disruption of mitochondrial function.
Concentration (µM) | Cell Viability (%) | Apoptosis Rate (%) |
---|---|---|
0 | 100 | 5 |
50 | 90 | 10 |
100 | 60 | 30 |
200 | 30 | 70 |
Case Study 2: Interaction with Proteins
Another investigation focused on the interaction of DNE with serum albumin. Using spectroscopic methods, researchers found that DNE binds to albumin with a dissociation constant indicating a moderate affinity. This interaction suggests potential implications for drug delivery systems.
Research Findings Summary
- Oxidative Stress : DNE induces oxidative stress in various cell types, leading to cellular damage and apoptosis .
- Protein Binding : The compound's ability to bind proteins may influence its pharmacokinetics and therapeutic applications .
- Potential Applications : Due to its reactivity and biological activity, DNE could be explored for applications in drug development or as a biochemical probe in research settings.
Q & A
Basic Research Questions
Q. What experimental methods are recommended for synthesizing and characterizing 1,1-dinitroethane?
- Synthesis : this compound can be synthesized via base-mediated reactions. For example, reacting potassium carbonate with 1,1,1-trinitroethane followed by acidification yields this compound .
- Characterization : Use NMR, IR, and mass spectrometry to confirm structure. For purity assessment, employ HPLC or GC-MS. Ensure proper documentation of spectral data (e.g., δ values in NMR) and cross-reference with literature .
Q. How can researchers resolve discrepancies between experimental and predicted thermodynamic data for this compound?
- Data Validation : Compare experimental enthalpy values (e.g., ΔHf = -87.2 kJ/mol) with group contribution models. Address large absolute errors (e.g., 35.16 kJ/mol discrepancy) by revisiting assumptions in computational methods, such as bond dissociation energy approximations or solvent effects .
- Reproducibility : Replicate experiments under controlled conditions (temperature, solvent purity) and validate instrumentation calibration .
Properties
IUPAC Name |
1,1-dinitroethane | |
---|---|---|
Source | PubChem | |
URL | https://pubchem.ncbi.nlm.nih.gov | |
Description | Data deposited in or computed by PubChem | |
InChI |
InChI=1S/C2H4N2O4/c1-2(3(5)6)4(7)8/h2H,1H3 | |
Source | PubChem | |
URL | https://pubchem.ncbi.nlm.nih.gov | |
Description | Data deposited in or computed by PubChem | |
InChI Key |
LKKHEZBRRGJBGH-UHFFFAOYSA-N | |
Source | PubChem | |
URL | https://pubchem.ncbi.nlm.nih.gov | |
Description | Data deposited in or computed by PubChem | |
Canonical SMILES |
CC([N+](=O)[O-])[N+](=O)[O-] | |
Source | PubChem | |
URL | https://pubchem.ncbi.nlm.nih.gov | |
Description | Data deposited in or computed by PubChem | |
Molecular Formula |
C2H4N2O4 | |
Source | PubChem | |
URL | https://pubchem.ncbi.nlm.nih.gov | |
Description | Data deposited in or computed by PubChem | |
DSSTOX Substance ID |
DTXSID80208704 | |
Record name | Ethane, 1,1-dinitro- | |
Source | EPA DSSTox | |
URL | https://comptox.epa.gov/dashboard/DTXSID80208704 | |
Description | DSSTox provides a high quality public chemistry resource for supporting improved predictive toxicology. | |
Molecular Weight |
120.06 g/mol | |
Source | PubChem | |
URL | https://pubchem.ncbi.nlm.nih.gov | |
Description | Data deposited in or computed by PubChem | |
CAS No. |
600-40-8 | |
Record name | 1,1-Dinitroethane | |
Source | CAS Common Chemistry | |
URL | https://commonchemistry.cas.org/detail?cas_rn=600-40-8 | |
Description | CAS Common Chemistry is an open community resource for accessing chemical information. Nearly 500,000 chemical substances from CAS REGISTRY cover areas of community interest, including common and frequently regulated chemicals, and those relevant to high school and undergraduate chemistry classes. This chemical information, curated by our expert scientists, is provided in alignment with our mission as a division of the American Chemical Society. | |
Explanation | The data from CAS Common Chemistry is provided under a CC-BY-NC 4.0 license, unless otherwise stated. | |
Record name | 1,1-Dinitroethane | |
Source | ChemIDplus | |
URL | https://pubchem.ncbi.nlm.nih.gov/substance/?source=chemidplus&sourceid=0000600408 | |
Description | ChemIDplus is a free, web search system that provides access to the structure and nomenclature authority files used for the identification of chemical substances cited in National Library of Medicine (NLM) databases, including the TOXNET system. | |
Record name | 1,1-DINITROETHANE | |
Source | DTP/NCI | |
URL | https://dtp.cancer.gov/dtpstandard/servlet/dwindex?searchtype=NSC&outputformat=html&searchlist=16150 | |
Description | The NCI Development Therapeutics Program (DTP) provides services and resources to the academic and private-sector research communities worldwide to facilitate the discovery and development of new cancer therapeutic agents. | |
Explanation | Unless otherwise indicated, all text within NCI products is free of copyright and may be reused without our permission. Credit the National Cancer Institute as the source. | |
Record name | Ethane, 1,1-dinitro- | |
Source | EPA DSSTox | |
URL | https://comptox.epa.gov/dashboard/DTXSID80208704 | |
Description | DSSTox provides a high quality public chemistry resource for supporting improved predictive toxicology. | |
Record name | 1,1-DINITROETHANE | |
Source | European Chemicals Agency (ECHA) | |
URL | https://echa.europa.eu/information-on-chemicals | |
Description | The European Chemicals Agency (ECHA) is an agency of the European Union which is the driving force among regulatory authorities in implementing the EU's groundbreaking chemicals legislation for the benefit of human health and the environment as well as for innovation and competitiveness. | |
Explanation | Use of the information, documents and data from the ECHA website is subject to the terms and conditions of this Legal Notice, and subject to other binding limitations provided for under applicable law, the information, documents and data made available on the ECHA website may be reproduced, distributed and/or used, totally or in part, for non-commercial purposes provided that ECHA is acknowledged as the source: "Source: European Chemicals Agency, http://echa.europa.eu/". Such acknowledgement must be included in each copy of the material. ECHA permits and encourages organisations and individuals to create links to the ECHA website under the following cumulative conditions: Links can only be made to webpages that provide a link to the Legal Notice page. | |
Record name | 1,1-DINITROETHANE | |
Source | FDA Global Substance Registration System (GSRS) | |
URL | https://gsrs.ncats.nih.gov/ginas/app/beta/substances/5CTY3X4E7X | |
Description | The FDA Global Substance Registration System (GSRS) enables the efficient and accurate exchange of information on what substances are in regulated products. Instead of relying on names, which vary across regulatory domains, countries, and regions, the GSRS knowledge base makes it possible for substances to be defined by standardized, scientific descriptions. | |
Explanation | Unless otherwise noted, the contents of the FDA website (www.fda.gov), both text and graphics, are not copyrighted. They are in the public domain and may be republished, reprinted and otherwise used freely by anyone without the need to obtain permission from FDA. Credit to the U.S. Food and Drug Administration as the source is appreciated but not required. | |
Retrosynthesis Analysis
AI-Powered Synthesis Planning: Our tool employs the Template_relevance Pistachio, Template_relevance Bkms_metabolic, Template_relevance Pistachio_ringbreaker, Template_relevance Reaxys, Template_relevance Reaxys_biocatalysis model, leveraging a vast database of chemical reactions to predict feasible synthetic routes.
One-Step Synthesis Focus: Specifically designed for one-step synthesis, it provides concise and direct routes for your target compounds, streamlining the synthesis process.
Accurate Predictions: Utilizing the extensive PISTACHIO, BKMS_METABOLIC, PISTACHIO_RINGBREAKER, REAXYS, REAXYS_BIOCATALYSIS database, our tool offers high-accuracy predictions, reflecting the latest in chemical research and data.
Strategy Settings
Precursor scoring | Relevance Heuristic |
---|---|
Min. plausibility | 0.01 |
Model | Template_relevance |
Template Set | Pistachio/Bkms_metabolic/Pistachio_ringbreaker/Reaxys/Reaxys_biocatalysis |
Top-N result to add to graph | 6 |
Feasible Synthetic Routes
Disclaimer and Information on In-Vitro Research Products
Please be aware that all articles and product information presented on BenchChem are intended solely for informational purposes. The products available for purchase on BenchChem are specifically designed for in-vitro studies, which are conducted outside of living organisms. In-vitro studies, derived from the Latin term "in glass," involve experiments performed in controlled laboratory settings using cells or tissues. It is important to note that these products are not categorized as medicines or drugs, and they have not received approval from the FDA for the prevention, treatment, or cure of any medical condition, ailment, or disease. We must emphasize that any form of bodily introduction of these products into humans or animals is strictly prohibited by law. It is essential to adhere to these guidelines to ensure compliance with legal and ethical standards in research and experimentation.