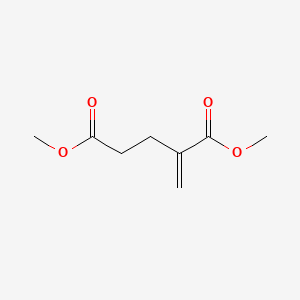
Dimethyl 2-methylenepentanedioate
Overview
Description
Dimethyl 2-methylenepentanedioate, also known as dimethyl 2-methyleneglutarate, is an organic compound with the molecular formula C₈H₁₂O₄. It is a dimethyl ester of 2-methylenepentanedioic acid and is characterized by the presence of a methylene group attached to the second carbon of the pentanedioate chain. This compound is of interest in various fields of chemistry due to its unique structure and reactivity.
Preparation Methods
Synthetic Routes and Reaction Conditions
Dimethyl 2-methylenepentanedioate can be synthesized through several methods. One common synthetic route involves the esterification of 2-methylenepentanedioic acid with methanol in the presence of an acid catalyst such as sulfuric acid. The reaction is typically carried out under reflux conditions to ensure complete esterification.
Another method involves the reaction of 2-methylenepentanedioic acid with dimethyl sulfate in the presence of a base such as sodium hydroxide. This method also results in the formation of this compound with high yield.
Industrial Production Methods
In an industrial setting, this compound can be produced through the catalytic hydrogenation of this compound precursors. This process involves the use of a metal catalyst such as palladium or platinum to facilitate the hydrogenation reaction. The reaction is carried out under high pressure and temperature to achieve optimal conversion rates.
Chemical Reactions Analysis
Types of Reactions
Dimethyl 2-methylenepentanedioate undergoes various chemical reactions, including:
Oxidation: The compound can be oxidized to form corresponding carboxylic acids or ketones.
Reduction: Reduction reactions can convert the ester groups to alcohols.
Substitution: The methylene group can undergo nucleophilic substitution reactions, leading to the formation of various substituted derivatives.
Common Reagents and Conditions
Oxidation: Common oxidizing agents include potassium permanganate and chromium trioxide.
Reduction: Reducing agents such as lithium aluminum hydride or sodium borohydride are used.
Substitution: Nucleophiles such as amines or thiols can be used for substitution reactions.
Major Products Formed
Oxidation: Products include 2-methylenepentanedioic acid and its derivatives.
Reduction: Products include 2-methylenepentanediol.
Substitution: Products include various substituted 2-methylenepentanedioates.
Scientific Research Applications
Dimethyl 2-methylenepentanedioate has several applications in scientific research:
Chemistry: It is used as a building block in organic synthesis for the preparation of more complex molecules.
Biology: The compound is studied for its potential biological activity and interactions with enzymes.
Medicine: Research is ongoing to explore its potential as a precursor for pharmaceutical compounds.
Industry: It is used in the production of polymers and other industrial chemicals.
Mechanism of Action
The mechanism of action of dimethyl 2-methylenepentanedioate involves its interaction with various molecular targets. The methylene group can participate in nucleophilic addition reactions, while the ester groups can undergo hydrolysis to form carboxylic acids. These reactions are facilitated by enzymes or chemical catalysts, leading to the formation of various products that exert biological or chemical effects.
Comparison with Similar Compounds
Similar Compounds
Dimethyl 2-methylpentanedioate: Similar structure but lacks the methylene group.
Dimethyl 2-methyleneglutarate: Another name for dimethyl 2-methylenepentanedioate.
Dimethyl 2-methylideneglutarate: Similar structure with slight variations in the positioning of functional groups.
Uniqueness
This compound is unique due to the presence of the methylene group, which imparts distinct reactivity and chemical properties. This makes it a valuable compound for various synthetic and industrial applications.
Properties
IUPAC Name |
dimethyl 2-methylidenepentanedioate | |
---|---|---|
Source | PubChem | |
URL | https://pubchem.ncbi.nlm.nih.gov | |
Description | Data deposited in or computed by PubChem | |
InChI |
InChI=1S/C8H12O4/c1-6(8(10)12-3)4-5-7(9)11-2/h1,4-5H2,2-3H3 | |
Source | PubChem | |
URL | https://pubchem.ncbi.nlm.nih.gov | |
Description | Data deposited in or computed by PubChem | |
InChI Key |
URLYMBMJTPDVEP-UHFFFAOYSA-N | |
Source | PubChem | |
URL | https://pubchem.ncbi.nlm.nih.gov | |
Description | Data deposited in or computed by PubChem | |
Canonical SMILES |
COC(=O)CCC(=C)C(=O)OC | |
Source | PubChem | |
URL | https://pubchem.ncbi.nlm.nih.gov | |
Description | Data deposited in or computed by PubChem | |
Molecular Formula |
C8H12O4 | |
Source | PubChem | |
URL | https://pubchem.ncbi.nlm.nih.gov | |
Description | Data deposited in or computed by PubChem | |
DSSTOX Substance ID |
DTXSID201288491 | |
Record name | 1,5-Dimethyl 2-methylenepentanedioate | |
Source | EPA DSSTox | |
URL | https://comptox.epa.gov/dashboard/DTXSID201288491 | |
Description | DSSTox provides a high quality public chemistry resource for supporting improved predictive toxicology. | |
Molecular Weight |
172.18 g/mol | |
Source | PubChem | |
URL | https://pubchem.ncbi.nlm.nih.gov | |
Description | Data deposited in or computed by PubChem | |
CAS No. |
5621-44-3 | |
Record name | 1,5-Dimethyl 2-methylenepentanedioate | |
Source | CAS Common Chemistry | |
URL | https://commonchemistry.cas.org/detail?cas_rn=5621-44-3 | |
Description | CAS Common Chemistry is an open community resource for accessing chemical information. Nearly 500,000 chemical substances from CAS REGISTRY cover areas of community interest, including common and frequently regulated chemicals, and those relevant to high school and undergraduate chemistry classes. This chemical information, curated by our expert scientists, is provided in alignment with our mission as a division of the American Chemical Society. | |
Explanation | The data from CAS Common Chemistry is provided under a CC-BY-NC 4.0 license, unless otherwise stated. | |
Record name | 1,5-Dimethyl 2-methylenepentanedioate | |
Source | EPA DSSTox | |
URL | https://comptox.epa.gov/dashboard/DTXSID201288491 | |
Description | DSSTox provides a high quality public chemistry resource for supporting improved predictive toxicology. | |
Synthesis routes and methods
Procedure details
Retrosynthesis Analysis
AI-Powered Synthesis Planning: Our tool employs the Template_relevance Pistachio, Template_relevance Bkms_metabolic, Template_relevance Pistachio_ringbreaker, Template_relevance Reaxys, Template_relevance Reaxys_biocatalysis model, leveraging a vast database of chemical reactions to predict feasible synthetic routes.
One-Step Synthesis Focus: Specifically designed for one-step synthesis, it provides concise and direct routes for your target compounds, streamlining the synthesis process.
Accurate Predictions: Utilizing the extensive PISTACHIO, BKMS_METABOLIC, PISTACHIO_RINGBREAKER, REAXYS, REAXYS_BIOCATALYSIS database, our tool offers high-accuracy predictions, reflecting the latest in chemical research and data.
Strategy Settings
Precursor scoring | Relevance Heuristic |
---|---|
Min. plausibility | 0.01 |
Model | Template_relevance |
Template Set | Pistachio/Bkms_metabolic/Pistachio_ringbreaker/Reaxys/Reaxys_biocatalysis |
Top-N result to add to graph | 6 |
Feasible Synthetic Routes
Disclaimer and Information on In-Vitro Research Products
Please be aware that all articles and product information presented on BenchChem are intended solely for informational purposes. The products available for purchase on BenchChem are specifically designed for in-vitro studies, which are conducted outside of living organisms. In-vitro studies, derived from the Latin term "in glass," involve experiments performed in controlled laboratory settings using cells or tissues. It is important to note that these products are not categorized as medicines or drugs, and they have not received approval from the FDA for the prevention, treatment, or cure of any medical condition, ailment, or disease. We must emphasize that any form of bodily introduction of these products into humans or animals is strictly prohibited by law. It is essential to adhere to these guidelines to ensure compliance with legal and ethical standards in research and experimentation.