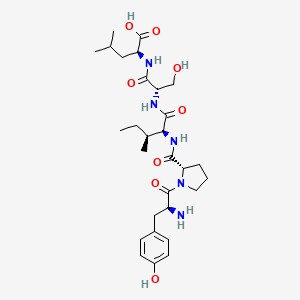
Gluten Exorphin C
Overview
Description
Gluten Exorphin C is a pentapeptide opioid peptide derived from the enzymatic digestion of gluten, a protein found in wheat. This peptide, with the sequence H-Tyr-Pro-Ile-Ser-Leu-OH, is known for its opioid-like activity, which means it can bind to opioid receptors in the body and mimic the effects of endogenous opioids . This compound has garnered interest due to its potential implications in various physiological processes and diseases, particularly those related to gluten sensitivity and celiac disease .
Mechanism of Action
Target of Action
Gluten Exorphin C is an opioid peptide derived from wheat gluten . It primarily targets the μ-opioid and δ-opioid receptors . These receptors are located in the central and peripheral nervous system, gastrointestinal tract, and some immune cells . They play a crucial role in pain modulation, mood regulation, and control of food intake .
Mode of Action
This compound interacts with its targets, the μ-opioid and δ-opioid receptors, by binding to these receptors . This binding mimics the action of endogenous opioids, leading to a series of downstream effects . The IC50 values for μ-opioid and δ-opioid activities are 40 μM and 13.5 μM, respectively .
Biochemical Pathways
Upon binding to the opioid receptors, this compound can modulate various biochemical pathways. It has been suggested that these peptides have a role in prolactin secretion, modification of brain neurotransmitter secretion, hormonal and gastrointestinal functions . The exact biochemical pathways affected by this compound are still under investigation.
Pharmacokinetics
It is known that these peptides are formed during the digestion of the gluten protein
Result of Action
The binding of this compound to the opioid receptors results in a variety of molecular and cellular effects. These include modulation of pain senses, observable behavior, learning, and memory processes in animal models . Moreover, it has been suggested that these peptides might play a role in various diseases and disorders, including celiac disease .
Action Environment
The action of this compound can be influenced by various environmental factors. For instance, the presence of gluten in the diet can affect the production and absorption of this compound . Additionally, individual susceptibility to gluten and casein allergies can influence the action of this compound
Biochemical Analysis
Biochemical Properties
Gluten Exorphin C plays a significant role in biochemical reactions by interacting with opioid receptors. It is known to inhibit the activity of both μ-opioid and δ-opioid receptors, with IC50 values of 40 μM and 13.5 μM, respectively . These interactions are crucial as they mimic the effects of endogenous opioids, influencing pain perception, mood, and other physiological functions. The peptide sequence of this compound is Tyr-Pro-Ile-Ser-Leu, and it is derived from the glutenin fraction of wheat gluten .
Cellular Effects
This compound affects various types of cells and cellular processes. It influences cell function by modulating cell signaling pathways, gene expression, and cellular metabolism. The interaction of this compound with opioid receptors can lead to changes in neurotransmitter release, impacting neuronal communication and potentially affecting mood and behavior . Additionally, this compound may influence gastrointestinal function by interacting with receptors in the gut, potentially affecting motility and secretion.
Molecular Mechanism
The mechanism of action of this compound involves its binding to opioid receptors, which are G-protein coupled receptors. Upon binding, this compound activates these receptors, leading to a cascade of intracellular events. This activation can result in the inhibition of adenylate cyclase, reducing the levels of cyclic adenosine monophosphate (cAMP) and subsequently altering the activity of protein kinase A (PKA). These changes can affect gene expression and other cellular processes . Additionally, this compound may modulate ion channel activity, influencing neuronal excitability and neurotransmitter release.
Temporal Effects in Laboratory Settings
In laboratory settings, the effects of this compound can change over time. The stability and degradation of this compound are important factors that influence its long-term effects on cellular function. Studies have shown that this compound can be stable under certain conditions, but it may degrade over time, leading to a decrease in its activity . Long-term exposure to this compound in in vitro or in vivo studies has been associated with changes in cellular function, including alterations in receptor sensitivity and gene expression.
Dosage Effects in Animal Models
The effects of this compound vary with different dosages in animal models. At low doses, this compound may exhibit beneficial effects, such as pain modulation and improved mood. At high doses, it can lead to adverse effects, including toxicity and negative impacts on behavior and physiological functions . Threshold effects have been observed, where the response to this compound changes significantly at certain dosage levels.
Metabolic Pathways
This compound is involved in metabolic pathways that include its interaction with enzymes and cofactors. It is metabolized by peptidases, which break down the peptide into smaller fragments. These metabolic processes can influence the levels of this compound and its activity in the body . Additionally, this compound may affect metabolic flux and the levels of other metabolites, potentially impacting overall metabolic homeostasis.
Transport and Distribution
This compound is transported and distributed within cells and tissues through interactions with transporters and binding proteins. These interactions can influence the localization and accumulation of this compound in specific tissues, such as the brain and gastrointestinal tract . The distribution of this compound is crucial for its physiological effects, as it determines the sites of action and the extent of its influence on various cellular processes.
Subcellular Localization
The subcellular localization of this compound is important for its activity and function. It may be directed to specific compartments or organelles through targeting signals or post-translational modifications. These localization mechanisms can influence the interactions of this compound with other biomolecules and its overall efficacy in modulating cellular processes . Understanding the subcellular distribution of this compound can provide insights into its precise role in cellular function and its potential therapeutic applications.
Preparation Methods
Synthetic Routes and Reaction Conditions: Gluten Exorphin C can be synthesized through enzymatic hydrolysis of wheat gluten. The process involves multiple steps:
Pepsin Digestion: Wheat gluten is first digested with pepsin in an acidic environment (pH 2.0) at 36°C for 17 hours.
Neutralization and Further Digestion: The pH is then adjusted to neutral (pH 7.0) using sodium hydroxide, followed by further digestion with trypsin and chymotrypsin at 36°C for 5 hours.
Industrial Production Methods: While the industrial production of this compound is not widely documented, it would likely involve scaling up the enzymatic hydrolysis process and optimizing the purification steps to ensure high yield and purity.
Chemical Reactions Analysis
Types of Reactions: Gluten Exorphin C primarily undergoes hydrolysis reactions during its formation. It can also participate in binding interactions with opioid receptors, which are not traditional chemical reactions but are crucial for its biological activity .
Common Reagents and Conditions:
Pepsin: Used in acidic conditions for initial digestion.
Trypsin and Chymotrypsin: Used in neutral conditions for further digestion.
Acetonitrile and Trifluoroacetic Acid: Used in HPLC for purification.
Major Products: The major product of these reactions is the pentapeptide this compound itself, with the sequence H-Tyr-Pro-Ile-Ser-Leu-OH .
Scientific Research Applications
Gluten Exorphin C has been studied for its potential roles in various fields:
Comparison with Similar Compounds
Gluten Exorphin A5: H-Gly-Tyr-Tyr-Pro-Thr-OH
Gluten Exorphin B4: H-Tyr-Gly-Gly-Trp-OH
Gluten Exorphin B5: H-Tyr-Gly-Gly-Trp-Leu-OH
Gliadorphin-7: H-Tyr-Pro-Gln-Pro-Gln-Pro-Phe-OH
Comparison: Gluten Exorphin C is unique among these peptides due to its specific sequence and its selectivity for the delta-opioid receptor . While other gluten exorphins also exhibit opioid activity, this compound’s distinct structure and receptor affinity make it particularly interesting for research into gluten-related disorders and opioid receptor interactions .
Properties
IUPAC Name |
(2S)-2-[[(2S)-2-[[(2S,3S)-2-[[(2S)-1-[(2S)-2-amino-3-(4-hydroxyphenyl)propanoyl]pyrrolidine-2-carbonyl]amino]-3-methylpentanoyl]amino]-3-hydroxypropanoyl]amino]-4-methylpentanoic acid | |
---|---|---|
Source | PubChem | |
URL | https://pubchem.ncbi.nlm.nih.gov | |
Description | Data deposited in or computed by PubChem | |
InChI |
InChI=1S/C29H45N5O8/c1-5-17(4)24(27(39)32-22(15-35)25(37)31-21(29(41)42)13-16(2)3)33-26(38)23-7-6-12-34(23)28(40)20(30)14-18-8-10-19(36)11-9-18/h8-11,16-17,20-24,35-36H,5-7,12-15,30H2,1-4H3,(H,31,37)(H,32,39)(H,33,38)(H,41,42)/t17-,20-,21-,22-,23-,24-/m0/s1 | |
Source | PubChem | |
URL | https://pubchem.ncbi.nlm.nih.gov | |
Description | Data deposited in or computed by PubChem | |
InChI Key |
QFRLEUJNZXTNTR-YYOLRRQBSA-N | |
Source | PubChem | |
URL | https://pubchem.ncbi.nlm.nih.gov | |
Description | Data deposited in or computed by PubChem | |
Canonical SMILES |
CCC(C)C(C(=O)NC(CO)C(=O)NC(CC(C)C)C(=O)O)NC(=O)C1CCCN1C(=O)C(CC2=CC=C(C=C2)O)N | |
Source | PubChem | |
URL | https://pubchem.ncbi.nlm.nih.gov | |
Description | Data deposited in or computed by PubChem | |
Isomeric SMILES |
CC[C@H](C)[C@@H](C(=O)N[C@@H](CO)C(=O)N[C@@H](CC(C)C)C(=O)O)NC(=O)[C@@H]1CCCN1C(=O)[C@H](CC2=CC=C(C=C2)O)N | |
Source | PubChem | |
URL | https://pubchem.ncbi.nlm.nih.gov | |
Description | Data deposited in or computed by PubChem | |
Molecular Formula |
C29H45N5O8 | |
Source | PubChem | |
URL | https://pubchem.ncbi.nlm.nih.gov | |
Description | Data deposited in or computed by PubChem | |
Molecular Weight |
591.7 g/mol | |
Source | PubChem | |
URL | https://pubchem.ncbi.nlm.nih.gov | |
Description | Data deposited in or computed by PubChem | |
Retrosynthesis Analysis
AI-Powered Synthesis Planning: Our tool employs the Template_relevance Pistachio, Template_relevance Bkms_metabolic, Template_relevance Pistachio_ringbreaker, Template_relevance Reaxys, Template_relevance Reaxys_biocatalysis model, leveraging a vast database of chemical reactions to predict feasible synthetic routes.
One-Step Synthesis Focus: Specifically designed for one-step synthesis, it provides concise and direct routes for your target compounds, streamlining the synthesis process.
Accurate Predictions: Utilizing the extensive PISTACHIO, BKMS_METABOLIC, PISTACHIO_RINGBREAKER, REAXYS, REAXYS_BIOCATALYSIS database, our tool offers high-accuracy predictions, reflecting the latest in chemical research and data.
Strategy Settings
Precursor scoring | Relevance Heuristic |
---|---|
Min. plausibility | 0.01 |
Model | Template_relevance |
Template Set | Pistachio/Bkms_metabolic/Pistachio_ringbreaker/Reaxys/Reaxys_biocatalysis |
Top-N result to add to graph | 6 |
Feasible Synthetic Routes
Disclaimer and Information on In-Vitro Research Products
Please be aware that all articles and product information presented on BenchChem are intended solely for informational purposes. The products available for purchase on BenchChem are specifically designed for in-vitro studies, which are conducted outside of living organisms. In-vitro studies, derived from the Latin term "in glass," involve experiments performed in controlled laboratory settings using cells or tissues. It is important to note that these products are not categorized as medicines or drugs, and they have not received approval from the FDA for the prevention, treatment, or cure of any medical condition, ailment, or disease. We must emphasize that any form of bodily introduction of these products into humans or animals is strictly prohibited by law. It is essential to adhere to these guidelines to ensure compliance with legal and ethical standards in research and experimentation.