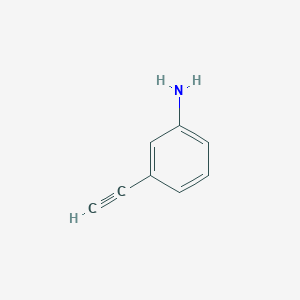
3-Ethynylaniline
Overview
Description
3-Ethynylaniline (CAS: 54060-30-9, molecular formula: C₈H₇N, molecular weight: 117.15 g/mol) is a substituted aniline derivative featuring an ethynyl group (-C≡CH) at the meta position of the aromatic ring. This structural motif enables diverse reactivity, including participation in Sonogashira coupling, Huisgen cycloaddition, and nucleophilic substitution reactions. It is a critical intermediate in synthesizing pharmaceuticals like erlotinib, a tyrosine kinase inhibitor used in non-small cell lung cancer treatment . Beyond oncology, this compound is employed in materials science for surface functionalization of silicon substrates and in antimicrobial drug discovery via in situ click chemistry .
Preparation Methods
Synthesis from m-Nitrocinnamic Acid via Bromination and Elimination
Bromination of m-Nitrocinnamic Acid
The synthesis begins with m-nitrocinnamic acid (C₉H₇NO₄), which undergoes bromination in glacial acetic acid at 60°C . Liquid bromine (0.11 mol) is added dropwise to a stirred solution of m-nitrocinnamic acid (0.10 mol) in acetic acid, yielding 2,3-dibromo-3-(3-nitrophenyl)propionic acid as a pale yellow solid (89.7% yield) . The reaction mechanism proceeds via electrophilic addition, with bromine attacking the α,β-unsaturated double bond of the cinnamic acid derivative .
Table 1: Reaction Conditions for Bromination Step
Parameter | Value |
---|---|
Starting Material | m-Nitrocinnamic acid (19.32 g) |
Bromine Equivalents | 1.1 mol |
Solvent | Glacial acetic acid (75 mL) |
Temperature | 60°C |
Reaction Time | 3 hours |
Yield | 89.7% |
Elimination Reaction with DBU
The dibrominated intermediate is treated with DBU (1:0.1–10 molar ratio) in tetrahydrofuran (THF) at 0–100°C for 1–24 hours . This step eliminates hydrogen bromide (HBr), forming 3-nitrophenylacetylene. The use of DBU, a strong organic base, facilitates dehydrohalogenation via an E2 mechanism . Purification via column chromatography (ethyl acetate/petroleum ether) affords the nitro-substituted alkyne in unquantified yield, though the overall process efficiency suggests >70% conversion .
Reduction of Nitro Group to Amine
The final step involves reducing 3-nitrophenylacetylene to 3-ethynylaniline using iron powder in a ethanol-water mixture (pH 5) at 60°C . Glacial acetic acid (14.7 mL) is added to a refluxing solution of 3-nitrophenylacetylene (14.6 g) and iron powder (14.7 g), achieving 85% yield after 7 hours . The nitro group is selectively reduced to an amine while preserving the alkyne functionality, as confirmed by NMR (δ 3.72 ppm for NH₂) and MS (m/z 117) .
Table 2: Reduction Step Optimization
Parameter | Value |
---|---|
Substrate | 3-Nitrophenylacetylene (14.6 g) |
Reducing Agent | Iron powder (14.7 g) |
Solvent System | Ethanol/water (100 mL) |
Temperature | 60°C (reflux) |
Reaction Time | 7 hours |
Yield | 85% |
Analytical Characterization and Physicochemical Properties
This compound is characterized by distinct spectroscopic and physical properties:
-
¹H-NMR (CDCl₃): δ 3.72 (s, 2H, NH₂), 6.30–7.25 (m, 4H, aromatic) .
-
EI-MS: m/z 117 (M⁺), with fragmentation peaks at m/z 93 and 77 .
Table 3: Physicochemical Properties of this compound
Property | Value |
---|---|
Molecular Weight | 117.15 g/mol |
Melting Point | 27°C |
Flash Point | 59°C |
Storage Conditions | Refrigerated |
Purity (GC) | ≥97.5% |
Applications in Organic Synthesis
This compound is pivotal in synthesizing fluorescent probes and pharmaceuticals. For example, it reacts with rhodamine B under HATU coupling to form lactam-based fluorophores, enabling bioimaging applications . Additionally, it serves as a precursor to erlotinib intermediates, highlighting its role in antitumor drug development .
Chemical Reactions Analysis
Types of Reactions: 3-Ethynylaniline undergoes various chemical reactions, including:
Oxidation: The compound can be oxidized to form corresponding quinones or other oxidized derivatives.
Reduction: Reduction of this compound can lead to the formation of 3-ethylaniline.
Common Reagents and Conditions:
Oxidation: Common oxidizing agents include potassium permanganate and chromium trioxide.
Reduction: Reducing agents like hydrogen gas with palladium catalyst or tin(II) chloride in hydrochloric acid are commonly used.
Major Products:
Oxidation: Quinones and other oxidized derivatives.
Reduction: 3-Ethylaniline.
Substitution: Various substituted derivatives depending on the electrophile used.
Scientific Research Applications
Pharmaceutical Applications
3-Ethynylaniline is primarily recognized for its role as a genotoxic impurity in the synthesis of certain pharmaceuticals, notably erlotinib, which is used in cancer treatment. The compound's presence necessitates rigorous analytical methods for quantification and quality control:
- Quantitative Analysis : A study developed a sensitive Liquid Chromatography-Tandem Mass Spectrometry (LC-MS/MS) method for the determination of this compound in erlotinib. This method demonstrated excellent linearity and recovery rates (96.26% to 100.64%) at low concentrations (as low as 0.6 ppm) .
- Impurity Monitoring : The detection of this compound as an impurity is critical due to its potential genotoxic effects. The LC-MS/MS method provides a reliable approach for monitoring this impurity during pharmaceutical manufacturing processes .
Analytical Chemistry Applications
In analytical chemistry, this compound serves as a reagent in various synthetic pathways and methodologies:
- Click Chemistry : The compound is utilized in click chemistry reactions, which are pivotal for synthesizing complex organic molecules efficiently. For instance, it has been employed to create novel polyamine ligands that exhibit DNA-damaging properties, demonstrating its utility in developing metallodrugs .
- Synthesis of Derivatives : this compound acts as a precursor in the synthesis of various derivatives, such as N-Boc-3-ethynylaniline and 3-ethynyl-N,N-dimethylaniline. These derivatives have applications in drug development and materials science .
Materials Science Applications
The compound is also significant in materials science, particularly in the development of new materials with specialized properties:
- Polymer Synthesis : Research indicates that this compound can be used to synthesize diacetylene-containing polymers, which are explored for their optical and electronic properties. These polymers have potential applications in creating transparent films and other advanced materials .
- Nanotechnology : In nanobiotechnology, this compound is being investigated for its role in developing drug delivery systems. Its functionalization can enhance the targeting capabilities of nanoparticles used for therapeutic applications .
Case Studies
- Erlotinib Synthesis :
- Development of Metallodrugs :
Mechanism of Action
The mechanism of action of 3-ethynylaniline and its derivatives often involves interactions with specific molecular targets and pathways. For instance, in medicinal chemistry, this compound derivatives may inhibit enzymes or receptors involved in disease pathways. The terminal alkyne group can also participate in click chemistry reactions, forming stable triazole linkages with azides, which is useful in bioconjugation and material science .
Comparison with Similar Compounds
Structural Analogs: Positional Isomerism and Electronic Effects
Ortho- and para-ethynylaniline isomers exhibit distinct reactivity compared to the meta isomer. In thermal grafting on hydrogenated silicon (100) surfaces:
- 3-Ethynylaniline reacts via the NH₂ group to form Si–N bonds due to resonance stabilization of the nucleophilic amine.
- 2- and 4-Ethynylaniline react via the ethynyl group to form Si–C bonds, driven by electron-withdrawing effects of the acetylene .
Table 1: Grafting Behavior of Ethynylaniline Isomers on Si(100)
Isomer | Reaction Pathway | Bond Formation | Key Resonance Effect |
---|---|---|---|
2-Ethynylaniline | Acetylene | Si–C | Electron-withdrawing acetylene |
This compound | NH₂ group | Si–N | Resonance-stabilized amine |
4-Ethynylaniline | Acetylene | Si–C | Electron-withdrawing acetylene |
Table 2: Toxicity Profiles of this compound Analogs
Compound | Scaffold | Substitution | CHI Score vs. Erlotinib | Key Observation |
---|---|---|---|---|
Erlotinib | Quinazoline | This compound | Baseline (1.0) | High EGFR potency |
28 | 3-Cyanoquinoline | This compound | 0.90 | 10% lower toxicity |
33 | 3-Cyanoquinoline | 3,4,5-Trimethoxyaniline | 1.05 | Similar CHI, improved selectivity |
36 | 3-Cyanoquinoline | Unsubstituted | 0.50 | Two-fold toxicity reduction |
Key Research Findings and Implications
- Pharmacological Trade-offs : While this compound’s removal in erlotinib analogs reduces dark toxicity, it compromises phototherapeutic indices (PI), underscoring its role in balancing efficacy and safety .
- Toxicity Modulation : Substituents on the aniline ring (e.g., halogens, methoxy groups) significantly alter CHI scores, guiding scaffold optimization in kinase inhibitor design .
- Synthetic Versatility : The ethynyl group’s reactivity enables diverse applications, from silicon surface modifications to PROTAC synthesis .
Biological Activity
3-Ethynylaniline, a compound with the molecular formula C₈H₇N, has garnered attention in various fields of biological research due to its potential therapeutic applications. This article reviews the biological activity of this compound, focusing on its synthesis, biological effects, and recent findings in pharmacological studies.
- Molecular Weight : 117.15 g/mol
- Boiling Point : 92–93 °C (2 mmHg)
- Melting Point : 27 °C
- Density : 1.04 g/cm³
Synthesis of this compound Derivatives
Research has shown that this compound serves as a precursor for synthesizing various biologically active compounds. For instance, derivatives synthesized from this compound have been evaluated for their potential in treating heart failure and other conditions.
Case Study: Heart Failure Treatment
A study aimed at synthesizing aniline derivatives from this compound assessed their biological activity against heart failure using an ischemia/reperfusion injury model. The results indicated that one of the synthesized compounds (compound 8) significantly reduced infarction area, suggesting its potential as a therapeutic agent for heart failure management .
Anticancer Properties
This compound derivatives have been explored for their anticancer activities. A notable study synthesized a series of 3-ethynyl-1H-indazoles, which exhibited low micromolar inhibition against key kinases involved in cancer progression, specifically targeting the PI3K pathway. The most active compound demonstrated significant anti-proliferative effects in human cancer cell cultures and three-dimensional tumor models .
The mechanism by which this compound derivatives exert their biological effects often involves modulation of signaling pathways critical for cell survival and proliferation. For example, compounds derived from this compound have been shown to inhibit PI3K, PDK1, and mTOR kinases, which are pivotal in oncogenic signaling . The structure-activity relationship (SAR) studies suggest that specific modifications to the ethynyl group can enhance inhibitory potency against these targets.
Summary of Findings
The following table summarizes key findings regarding the biological activity of this compound and its derivatives:
Q & A
Basic Research Questions
Q. What are the common synthetic routes for 3-ethynylaniline, and how are intermediates characterized?
- Methodological Answer : this compound is synthesized via Sonogashira coupling or nucleophilic substitution. For example, it reacts with benzoic acid derivatives (e.g., ethyl 2-azidoacetate) under Pd-catalyzed conditions (PdCl₂(PPh₃)₂, TBAF, 85°C) to form intermediates like compounds 5a–5i . Characterization involves NMR, LC-MS, and elemental analysis. Yield optimization (e.g., 67.88% for 5a) depends on stoichiometry and reaction time .
Q. How does this compound function as a pharmacophore in EGFR-targeted conjugates?
- Methodological Answer : In erlotinib-Pc conjugates, this compound serves as a linker between the drug and photosensitizer. Molecular docking studies (e.g., binding energy = −9.62 kcal mol⁻¹) show its minimal impact on EGFR binding compared to analogues lacking this group. However, its removal increases dark toxicity (IC₅₀ shifts from >100 μM to ~10 μM), highlighting its role in reducing off-target effects .
Q. What analytical techniques validate the purity and structure of this compound derivatives?
- Methodological Answer : High-resolution mass spectrometry (HR-MS) and X-ray photoelectron spectroscopy (XPS) confirm structural integrity. For silicon surface grafting, XPS detects Si–N bond formation (398.5 eV binding energy) in meta-substituted derivatives, while AFM quantifies surface roughness (<1 nm variation) .
Advanced Research Questions
Q. How do resonance effects in this compound influence its reactivity in surface grafting?
- Methodological Answer : The meta-substituted NH₂ group exhibits nucleophilic grafting on hydrogenated Si(100) surfaces, forming Si–N bonds due to resonance stabilization (electron donation from NH₂ to acetylene). In contrast, ortho/para isomers react via acetylene (Si–C bonds). Protected derivatives (e.g., tert-butoxycarbonyl) confirm this mechanism by forcing acetylene reactivity .
Q. What contradictions arise in computational vs. experimental data on this compound’s role in photodynamic therapy (PDT)?
- Methodological Answer : Docking studies predict retained EGFR binding after removing this compound (ΔG = −9.97 vs. −9.62 kcal mol⁻¹), but experimental PI values (phototoxicity index) drop from >5200 to <2000 due to increased dark toxicity. This discrepancy highlights the need for combined computational/experimental validation of linker effects .
Q. How does this compound enhance selectivity in cancer cell targeting?
- Methodological Answer : Conjugates like SiPc–erlotinib show 12-fold higher uptake in HepG2 (high EGFR) vs. HELF (low EGFR) cells via fluorescence intensity assays. Removing this compound reduces selectivity, confirming its role in stabilizing ligand-receptor interactions .
Properties
IUPAC Name |
3-ethynylaniline | |
---|---|---|
Source | PubChem | |
URL | https://pubchem.ncbi.nlm.nih.gov | |
Description | Data deposited in or computed by PubChem | |
InChI |
InChI=1S/C8H7N/c1-2-7-4-3-5-8(9)6-7/h1,3-6H,9H2 | |
Source | PubChem | |
URL | https://pubchem.ncbi.nlm.nih.gov | |
Description | Data deposited in or computed by PubChem | |
InChI Key |
NNKQLUVBPJEUOR-UHFFFAOYSA-N | |
Source | PubChem | |
URL | https://pubchem.ncbi.nlm.nih.gov | |
Description | Data deposited in or computed by PubChem | |
Canonical SMILES |
C#CC1=CC(=CC=C1)N | |
Source | PubChem | |
URL | https://pubchem.ncbi.nlm.nih.gov | |
Description | Data deposited in or computed by PubChem | |
Molecular Formula |
C8H7N | |
Source | PubChem | |
URL | https://pubchem.ncbi.nlm.nih.gov | |
Description | Data deposited in or computed by PubChem | |
DSSTOX Substance ID |
DTXSID30885746 | |
Record name | Benzenamine, 3-ethynyl- | |
Source | EPA DSSTox | |
URL | https://comptox.epa.gov/dashboard/DTXSID30885746 | |
Description | DSSTox provides a high quality public chemistry resource for supporting improved predictive toxicology. | |
Molecular Weight |
117.15 g/mol | |
Source | PubChem | |
URL | https://pubchem.ncbi.nlm.nih.gov | |
Description | Data deposited in or computed by PubChem | |
CAS No. |
54060-30-9 | |
Record name | 3-Ethynylaniline | |
Source | CAS Common Chemistry | |
URL | https://commonchemistry.cas.org/detail?cas_rn=54060-30-9 | |
Description | CAS Common Chemistry is an open community resource for accessing chemical information. Nearly 500,000 chemical substances from CAS REGISTRY cover areas of community interest, including common and frequently regulated chemicals, and those relevant to high school and undergraduate chemistry classes. This chemical information, curated by our expert scientists, is provided in alignment with our mission as a division of the American Chemical Society. | |
Explanation | The data from CAS Common Chemistry is provided under a CC-BY-NC 4.0 license, unless otherwise stated. | |
Record name | 3-Ethynylaniline | |
Source | ChemIDplus | |
URL | https://pubchem.ncbi.nlm.nih.gov/substance/?source=chemidplus&sourceid=0054060309 | |
Description | ChemIDplus is a free, web search system that provides access to the structure and nomenclature authority files used for the identification of chemical substances cited in National Library of Medicine (NLM) databases, including the TOXNET system. | |
Record name | Benzenamine, 3-ethynyl- | |
Source | EPA Chemicals under the TSCA | |
URL | https://www.epa.gov/chemicals-under-tsca | |
Description | EPA Chemicals under the Toxic Substances Control Act (TSCA) collection contains information on chemicals and their regulations under TSCA, including non-confidential content from the TSCA Chemical Substance Inventory and Chemical Data Reporting. | |
Record name | Benzenamine, 3-ethynyl- | |
Source | EPA DSSTox | |
URL | https://comptox.epa.gov/dashboard/DTXSID30885746 | |
Description | DSSTox provides a high quality public chemistry resource for supporting improved predictive toxicology. | |
Record name | 3-ethynylaniline | |
Source | European Chemicals Agency (ECHA) | |
URL | https://echa.europa.eu/substance-information/-/substanceinfo/100.053.568 | |
Description | The European Chemicals Agency (ECHA) is an agency of the European Union which is the driving force among regulatory authorities in implementing the EU's groundbreaking chemicals legislation for the benefit of human health and the environment as well as for innovation and competitiveness. | |
Explanation | Use of the information, documents and data from the ECHA website is subject to the terms and conditions of this Legal Notice, and subject to other binding limitations provided for under applicable law, the information, documents and data made available on the ECHA website may be reproduced, distributed and/or used, totally or in part, for non-commercial purposes provided that ECHA is acknowledged as the source: "Source: European Chemicals Agency, http://echa.europa.eu/". Such acknowledgement must be included in each copy of the material. ECHA permits and encourages organisations and individuals to create links to the ECHA website under the following cumulative conditions: Links can only be made to webpages that provide a link to the Legal Notice page. | |
Record name | 3-ETHYNYLANILINE | |
Source | FDA Global Substance Registration System (GSRS) | |
URL | https://gsrs.ncats.nih.gov/ginas/app/beta/substances/DU8GFZ5KGE | |
Description | The FDA Global Substance Registration System (GSRS) enables the efficient and accurate exchange of information on what substances are in regulated products. Instead of relying on names, which vary across regulatory domains, countries, and regions, the GSRS knowledge base makes it possible for substances to be defined by standardized, scientific descriptions. | |
Explanation | Unless otherwise noted, the contents of the FDA website (www.fda.gov), both text and graphics, are not copyrighted. They are in the public domain and may be republished, reprinted and otherwise used freely by anyone without the need to obtain permission from FDA. Credit to the U.S. Food and Drug Administration as the source is appreciated but not required. | |
Synthesis routes and methods I
Procedure details
Synthesis routes and methods II
Procedure details
Synthesis routes and methods III
Procedure details
Synthesis routes and methods IV
Procedure details
Retrosynthesis Analysis
AI-Powered Synthesis Planning: Our tool employs the Template_relevance Pistachio, Template_relevance Bkms_metabolic, Template_relevance Pistachio_ringbreaker, Template_relevance Reaxys, Template_relevance Reaxys_biocatalysis model, leveraging a vast database of chemical reactions to predict feasible synthetic routes.
One-Step Synthesis Focus: Specifically designed for one-step synthesis, it provides concise and direct routes for your target compounds, streamlining the synthesis process.
Accurate Predictions: Utilizing the extensive PISTACHIO, BKMS_METABOLIC, PISTACHIO_RINGBREAKER, REAXYS, REAXYS_BIOCATALYSIS database, our tool offers high-accuracy predictions, reflecting the latest in chemical research and data.
Strategy Settings
Precursor scoring | Relevance Heuristic |
---|---|
Min. plausibility | 0.01 |
Model | Template_relevance |
Template Set | Pistachio/Bkms_metabolic/Pistachio_ringbreaker/Reaxys/Reaxys_biocatalysis |
Top-N result to add to graph | 6 |
Feasible Synthetic Routes
Disclaimer and Information on In-Vitro Research Products
Please be aware that all articles and product information presented on BenchChem are intended solely for informational purposes. The products available for purchase on BenchChem are specifically designed for in-vitro studies, which are conducted outside of living organisms. In-vitro studies, derived from the Latin term "in glass," involve experiments performed in controlled laboratory settings using cells or tissues. It is important to note that these products are not categorized as medicines or drugs, and they have not received approval from the FDA for the prevention, treatment, or cure of any medical condition, ailment, or disease. We must emphasize that any form of bodily introduction of these products into humans or animals is strictly prohibited by law. It is essential to adhere to these guidelines to ensure compliance with legal and ethical standards in research and experimentation.