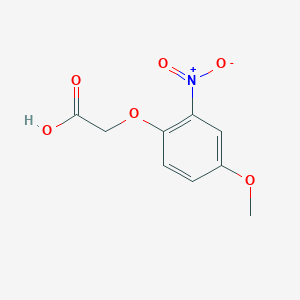
2-(4-Methoxy-2-nitrophenoxy)acetic acid
- Click on QUICK INQUIRY to receive a quote from our team of experts.
- With the quality product at a COMPETITIVE price, you can focus more on your research.
Overview
Description
2-(4-Methoxy-2-nitrophenoxy)acetic acid is an organic compound with the molecular formula C9H9NO5 It is characterized by the presence of a methoxy group, a nitro group, and a phenoxyacetic acid moiety
Preparation Methods
Synthetic Routes and Reaction Conditions
The synthesis of 2-(4-Methoxy-2-nitrophenoxy)acetic acid typically involves the nitration of 4-methoxyphenol followed by the reaction with chloroacetic acid. The nitration process introduces the nitro group into the aromatic ring, and the subsequent reaction with chloroacetic acid forms the phenoxyacetic acid structure. The reaction conditions often include the use of concentrated sulfuric acid and nitric acid for nitration, followed by neutralization and esterification steps .
Industrial Production Methods
Industrial production methods for this compound may involve similar synthetic routes but on a larger scale. The use of continuous flow reactors and optimized reaction conditions can enhance yield and purity. Catalysts and solvents are selected to ensure efficient conversion and minimal by-products .
Chemical Reactions Analysis
Types of Reactions
2-(4-Methoxy-2-nitrophenoxy)acetic acid can undergo various chemical reactions, including:
Oxidation: The methoxy group can be oxidized to form corresponding aldehydes or carboxylic acids.
Reduction: The nitro group can be reduced to an amino group under hydrogenation conditions.
Substitution: The aromatic ring can undergo electrophilic substitution reactions, such as halogenation or nitration.
Common Reagents and Conditions
Oxidation: Common oxidizing agents include potassium permanganate (KMnO4) and chromium trioxide (CrO3).
Reduction: Hydrogen gas (H2) in the presence of a palladium catalyst (Pd/C) is commonly used for nitro group reduction.
Substitution: Halogenation can be achieved using halogens (Cl2, Br2) in the presence of a Lewis acid catalyst.
Major Products
Oxidation: Formation of 4-methoxy-2-nitrobenzoic acid.
Reduction: Formation of 2-(4-methoxy-2-aminophenoxy)acetic acid.
Substitution: Formation of halogenated derivatives of the original compound.
Scientific Research Applications
2-(4-Methoxy-2-nitrophenoxy)acetic acid has several scientific research applications:
Chemistry: Used as an intermediate in the synthesis of more complex organic molecules.
Biology: Investigated for its potential as a biochemical probe to study enzyme interactions and metabolic pathways.
Medicine: Explored for its potential therapeutic properties, including anti-inflammatory and anticancer activities.
Industry: Utilized in the production of specialty chemicals and materials.
Mechanism of Action
The mechanism of action of 2-(4-Methoxy-2-nitrophenoxy)acetic acid involves its interaction with specific molecular targets. The nitro group can undergo bioreduction to form reactive intermediates that interact with cellular components, leading to various biological effects. The methoxy group may enhance the compound’s lipophilicity, facilitating its cellular uptake and distribution .
Comparison with Similar Compounds
Similar Compounds
2-(4-Methoxyphenoxy)acetic acid: Lacks the nitro group, resulting in different chemical reactivity and biological activity.
2-(4-Nitrophenoxy)acetic acid: Lacks the methoxy group, affecting its solubility and interaction with biological targets.
4-Methoxy-2-nitrobenzoic acid: Similar structure but lacks the phenoxyacetic acid moiety, leading to different applications and properties.
Uniqueness
2-(4-Methoxy-2-nitrophenoxy)acetic acid is unique due to the combination of the methoxy, nitro, and phenoxyacetic acid groups, which confer distinct chemical reactivity and potential biological activities. This combination makes it a valuable compound for various research and industrial applications .
Biological Activity
2-(4-Methoxy-2-nitrophenoxy)acetic acid is a compound of interest in medicinal chemistry due to its potential biological activities, including antimicrobial and anti-inflammatory properties. This article provides a detailed examination of its biological activity, supported by research findings, data tables, and case studies.
Chemical Structure and Properties
The compound features a methoxy group and a nitro group on the phenyl ring, which contribute to its unique chemical reactivity and biological activity. The general structure can be represented as follows:
Chemical Characteristics
- Molecular Weight : 209.21 g/mol
- Solubility : Soluble in polar solvents due to the presence of the methoxy and carboxylic acid groups.
Antimicrobial Properties
Recent studies have indicated that this compound exhibits significant antimicrobial activity against various bacterial strains. For instance, it has been tested against Gram-positive and Gram-negative bacteria, showing varying degrees of inhibition.
Table 1: Antimicrobial Activity
Bacterial Strain | Minimum Inhibitory Concentration (MIC) |
---|---|
Staphylococcus aureus | 32 µg/mL |
Escherichia coli | 64 µg/mL |
Pseudomonas aeruginosa | 128 µg/mL |
Anti-inflammatory Effects
The compound has also been investigated for its anti-inflammatory properties. In vitro studies demonstrated that it can inhibit the production of pro-inflammatory cytokines such as TNF-α and IL-6 in macrophage cell lines.
Case Study: Inhibition of Cytokine Production
In a controlled study, macrophages treated with 50 µg/mL of this compound showed a reduction in TNF-α levels by approximately 40% compared to untreated controls.
The mechanism through which this compound exerts its biological effects involves:
- Reduction of Nitro Group : The nitro group can be reduced to form reactive intermediates that interact with cellular components.
- Influence on Enzyme Activity : The methoxy group may enhance the compound's ability to bind to specific enzymes or receptors involved in inflammatory pathways.
Potential Applications in Medicine
Given its biological activities, this compound is being explored as a potential therapeutic agent for conditions such as:
- Infections : Due to its antimicrobial properties.
- Inflammatory Diseases : As a potential treatment for chronic inflammatory conditions.
Synthesis and Characterization
Research has established efficient synthetic routes for producing this compound. The synthesis typically involves the reaction of appropriate nitro-substituted phenols with acetic acid derivatives under controlled conditions.
Comparative Studies
Comparative studies with similar compounds highlight the unique properties of this compound. For example, compounds like 4-Nitrophenylacetic acid lack the methoxy group, which is crucial for enhancing solubility and bioactivity.
Table 2: Comparison with Similar Compounds
Compound | Key Features | Biological Activity |
---|---|---|
4-Nitrophenylacetic acid | Lacks methoxy group | Limited antimicrobial activity |
2-Methoxy-4-nitrobenzoic acid | Different positioning on benzene ring | Moderate anti-inflammatory effects |
2-(4-Methoxyphenyl)acetic acid | Lacks nitro group | Lower overall activity |
Properties
Molecular Formula |
C9H9NO6 |
---|---|
Molecular Weight |
227.17 g/mol |
IUPAC Name |
2-(4-methoxy-2-nitrophenoxy)acetic acid |
InChI |
InChI=1S/C9H9NO6/c1-15-6-2-3-8(16-5-9(11)12)7(4-6)10(13)14/h2-4H,5H2,1H3,(H,11,12) |
InChI Key |
BUTBJANGJJMOEJ-UHFFFAOYSA-N |
Canonical SMILES |
COC1=CC(=C(C=C1)OCC(=O)O)[N+](=O)[O-] |
Origin of Product |
United States |
Disclaimer and Information on In-Vitro Research Products
Please be aware that all articles and product information presented on BenchChem are intended solely for informational purposes. The products available for purchase on BenchChem are specifically designed for in-vitro studies, which are conducted outside of living organisms. In-vitro studies, derived from the Latin term "in glass," involve experiments performed in controlled laboratory settings using cells or tissues. It is important to note that these products are not categorized as medicines or drugs, and they have not received approval from the FDA for the prevention, treatment, or cure of any medical condition, ailment, or disease. We must emphasize that any form of bodily introduction of these products into humans or animals is strictly prohibited by law. It is essential to adhere to these guidelines to ensure compliance with legal and ethical standards in research and experimentation.