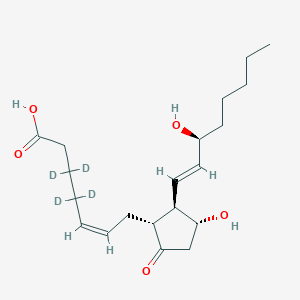
Prostaglandin E2-d4
Overview
Description
Prostaglandin E2-d4 is a deuterated form of Prostaglandin E2, containing four deuterium atoms at the 3, 3’, 4, and 4’ positions. It is primarily used as an internal standard for the quantification of Prostaglandin E2 by gas chromatography or liquid chromatography-mass spectrometry. Prostaglandin E2 is one of the primary cyclooxygenase products of arachidonic acid and is widely investigated for its role in various physiological processes, including inflammation, fertility, and immune modulation .
Mechanism of Action
Prostaglandin E2-d4, also known as (Z)-3,3,4,4-tetradeuterio-7-[(1R,2R,3R)-3-hydroxy-2-[(E,3S)-3-hydroxyoct-1-enyl]-5-oxocyclopentyl]hept-5-enoic acid, is a deuterium-labeled variant of Prostaglandin E2 (PGE2). It plays a significant role in various physiological processes and has a wide range of effects on the body .
Target of Action
The primary targets of this compound are the four G protein-coupled receptors (GPCRs) designated EP1, EP2, EP3, and EP4 . These receptors are expressed in various combinations on the plasma membrane of cells . PGE2 modulates several key immunological processes including the activation, migration, and cytokine production of different immune cells such as dendritic cells (DCs), macrophages, and T lymphocytes .
Mode of Action
This compound interacts with its targets, the EP receptors, leading to various downstream effects. Both EP2 and EP4 signaling lead to an elevation of intracellular cyclic adenosine monophosphate (cAMP) levels through the stimulating Gαs protein . EP4 also couples to the inhibitory Gαi protein to decrease the production of cAMP . This interaction with its targets results in changes in cell function, including the dissolution of adhesion structures called podosomes, which is a first and essential step in DC maturation .
Biochemical Pathways
The activation of the EP receptors by this compound affects several biochemical pathways. The elevation of cAMP levels is a key event in these pathways . EP2 and EP4 signaling leads to distinct cAMP production profiles, with EP4 inducing a transient cAMP response and EP2 inducing a sustained cAMP response only at high PGE2 levels . This modulation of cAMP levels influences various cellular processes, including the activation, migration, and cytokine production of immune cells .
Pharmacokinetics
It is known that the compound’s activity is influenced by its interaction with the ep receptors and the resulting changes in camp levels .
Result of Action
The action of this compound results in a range of molecular and cellular effects. It influences inflammation, fertility and parturition, gastric mucosal integrity, and immune modulation . Its effects on immune cells, such as DCs and macrophages, include changes in activation, migration, and cytokine production .
Action Environment
The action, efficacy, and stability of this compound can be influenced by various environmental factors. For instance, the spatiotemporal organization of GPCRs within the cell membrane allows these receptors to elicit fine-tuned cellular responses to different ligands . Additionally, the presence of other signaling molecules and the overall state of the cell can impact the action of this compound.
Biochemical Analysis
Biochemical Properties
Prostaglandin E2-d4 is involved in several biochemical reactions, primarily through its interaction with specific enzymes and receptors. It interacts with cyclooxygenase (COX) enzymes, which catalyze the conversion of arachidonic acid to Prostaglandin E2 . These interactions lead to various downstream effects, including the modulation of cyclic adenosine monophosphate (cAMP) levels and the activation of protein kinase A (PKA) signaling pathways .
Cellular Effects
This compound exerts significant effects on various cell types and cellular processes. In immune cells such as macrophages and dendritic cells, this compound modulates the function of these cells through the activation of EP2 and EP4 receptors . This activation leads to changes in cAMP levels, which in turn influence cell signaling pathways and gene expression . In reproductive cells, this compound plays a role in ovulation, implantation, and pregnancy by interacting with EP receptors . Additionally, this compound affects smooth muscle cells by regulating their contraction and relaxation .
Molecular Mechanism
The molecular mechanism of action of this compound involves its binding to EP receptors, leading to the activation of downstream signaling pathways. Upon binding to EP2 and EP4 receptors, this compound induces the production of cAMP, which activates PKA . This activation results in the phosphorylation of various target proteins, ultimately leading to changes in gene expression and cellular function . Additionally, this compound can modulate the activity of other signaling pathways, such as the phosphoinositide 3-kinase (PI3K)/Akt pathway, by interacting with EP receptors .
Temporal Effects in Laboratory Settings
In laboratory settings, the effects of this compound can vary over time. The stability of this compound allows for consistent results in biochemical assays . Its effects on cellular function may change over time due to factors such as receptor desensitization and degradation . Long-term studies have shown that this compound can influence cellular processes such as inflammation and immune response over extended periods .
Dosage Effects in Animal Models
The effects of this compound in animal models are dose-dependent. At low doses, this compound can enhance immune responses and promote tissue repair . At high doses, it may lead to adverse effects such as inflammation and tissue damage . Studies have shown that the optimal dosage of this compound varies depending on the specific animal model and the desired outcome .
Metabolic Pathways
This compound is involved in the arachidonic acid metabolic pathway. It is synthesized from arachidonic acid through the action of COX enzymes and Prostaglandin E synthase . This compound can also be metabolized into various downstream products, which may have distinct biological activities . The regulation of these metabolic pathways is crucial for maintaining the balance of this compound levels in the body .
Transport and Distribution
This compound is transported and distributed within cells and tissues through specific transporters and binding proteins. Multidrug resistance-associated protein 4 (MRP4) and Prostaglandin transporter (PGT) are involved in the transport of this compound across cellular membranes . These transporters facilitate the movement of this compound to its target sites, where it can exert its biological effects . Additionally, this compound can bind to plasma proteins, which may influence its distribution and availability in the body .
Subcellular Localization
The subcellular localization of this compound is primarily determined by its interactions with specific receptors and enzymes. This compound is localized in the cytosol and can be enriched in the perinuclear region . This localization is influenced by its binding to EP receptors and its involvement in the synthesis of Prostaglandin E2 . Additionally, this compound can be found in various cellular compartments, including the Golgi apparatus and plasma membrane, where it participates in signaling and metabolic processes .
Preparation Methods
Synthetic Routes and Reaction Conditions: The synthesis of Prostaglandin E2-d4 involves the incorporation of deuterium atoms into the Prostaglandin E2 molecule. This can be achieved through various methods, including the use of deuterated reagents or solvents during the synthesis process. The specific synthetic route may vary depending on the desired isotopic purity and the availability of deuterated starting materials.
Industrial Production Methods: Industrial production of this compound typically involves large-scale synthesis using deuterated reagents and solvents. The process may include multiple steps of purification and quality control to ensure the isotopic purity and chemical stability of the final product. The use of advanced analytical techniques, such as nuclear magnetic resonance spectroscopy and mass spectrometry, is essential for verifying the isotopic composition and purity of this compound .
Chemical Reactions Analysis
Types of Reactions: Prostaglandin E2-d4 undergoes various chemical reactions, including oxidation, reduction, and substitution. These reactions are influenced by the presence of deuterium atoms, which can affect the reaction kinetics and product distribution.
Common Reagents and Conditions:
Oxidation: Common oxidizing agents, such as potassium permanganate or chromium trioxide, can be used to oxidize this compound. The reaction conditions typically involve acidic or neutral pH and controlled temperature to prevent over-oxidation.
Reduction: Reducing agents, such as sodium borohydride or lithium aluminum hydride, can be used to reduce this compound. The reaction conditions may include anhydrous solvents and low temperatures to ensure selective reduction.
Substitution: Substitution reactions involving this compound can be carried out using various nucleophiles or electrophiles. The reaction conditions depend on the nature of the substituent and the desired product.
Major Products: The major products formed from these reactions depend on the specific reaction conditions and reagents used. For example, oxidation of this compound may yield various oxidized derivatives, while reduction may produce reduced forms of the compound .
Scientific Research Applications
Prostaglandin E2-d4 has a wide range of scientific research applications, including:
Chemistry: Used as an internal standard in analytical chemistry for the quantification of Prostaglandin E2 in complex biological samples.
Biology: Investigated for its role in various biological processes, including inflammation, immune response, and cell signaling.
Medicine: Studied for its potential therapeutic applications in conditions such as inflammation, pain, and cardiovascular diseases.
Comparison with Similar Compounds
Prostaglandin E2: The non-deuterated form of Prostaglandin E2, widely studied for its physiological and pharmacological effects.
Prostaglandin E1: Another member of the prostaglandin family, known for its vasodilatory and anti-inflammatory properties.
Prostaglandin F2α: Involved in various reproductive processes and studied for its role in smooth muscle contraction.
Uniqueness: Prostaglandin E2-d4 is unique due to the presence of deuterium atoms, which provide distinct advantages in analytical applications. The deuterium atoms enhance the stability and detectability of the compound in mass spectrometry, making it an ideal internal standard for quantitative analysis .
Biological Activity
Prostaglandin E2-d4 (PGE2-d4) is a deuterated form of Prostaglandin E2 (PGE2), a lipid mediator involved in various physiological processes, including inflammation, immune response, and tissue repair. This article delves into the biological activity of PGE2-d4, highlighting its mechanisms of action, receptor interactions, and implications in health and disease.
Overview of Prostaglandin E2
PGE2 is synthesized from arachidonic acid through the cyclooxygenase (COX) pathway, primarily by the enzymes COX-1 and COX-2. It exerts its effects by binding to four distinct receptors: EP1, EP2, EP3, and EP4, each mediating different biological responses. The diverse actions of PGE2 include modulation of inflammation, pain perception, vascular tone regulation, and involvement in reproductive processes .
Biological Activity of PGE2-d4
PGE2-d4 serves as an internal standard for quantifying PGE2 in various biological samples using mass spectrometry techniques. Its biological activity mirrors that of PGE2 but allows for precise tracking and measurement due to the incorporation of deuterium . The following sections explore its specific biological activities.
Inflammatory Response
PGE2 plays a crucial role in the inflammatory response by promoting vasodilation and increasing vascular permeability. It enhances the recruitment of immune cells to sites of injury or infection. Studies have shown that PGE2 can modulate the activity of macrophages and neutrophils, influencing their migration and cytokine production .
Table 1: Effects of PGE2 on Inflammation
Effect | Mechanism |
---|---|
Vasodilation | Activation of EP2 and EP4 receptors |
Increased vascular permeability | EP3 receptor activation |
Immune cell recruitment | Modulation of chemokine release |
Tissue Repair and Regeneration
Recent research indicates that PGE2 is involved in tissue repair mechanisms. It promotes the proliferation and differentiation of stem cells into functional tissue cells. For instance, studies have demonstrated that PGE2 signaling through the EP2 receptor enhances cardiac stem cell differentiation post-myocardial infarction .
Case Study: Cardiac Regeneration
In a study involving young mice with induced myocardial infarction, activation of COX-2/PGE2/EP2 signaling was found to significantly enhance cardiac stem cell differentiation into cardiomyocytes, suggesting a potential therapeutic role for PGE2 in cardiac repair .
Cancer Progression
PGE2 has been implicated in cancer biology, where it can promote tumor growth and metastasis. It influences tumor microenvironments by modulating immune responses and enhancing angiogenesis. Research has shown that PGE2 can increase the differentiation of myeloid-derived suppressor cells (MDSCs), which are known to suppress anti-tumor immunity .
Table 2: Role of PGE2 in Cancer
Function | Impact on Cancer |
---|---|
Tumor growth promotion | Increases cell proliferation |
Metastasis enhancement | Modulates MDSC differentiation |
Angiogenesis induction | Promotes blood vessel formation |
Receptor Interactions
The biological effects of PGE2-d4 are mediated through its interaction with specific prostaglandin receptors:
- EP1 : Involved in pain sensation and smooth muscle contraction.
- EP2 : Promotes vasodilation and enhances cellular proliferation.
- EP3 : Regulates fever response and inhibits gastric acid secretion.
- EP4 : Plays a role in immune modulation and inflammation resolution.
The affinity constants (Kd) for these receptors vary from 1-10 nM depending on the receptor subtype, indicating a strong interaction between PGE2-d4 and its receptors .
Properties
IUPAC Name |
(Z)-3,3,4,4-tetradeuterio-7-[(1R,2R,3R)-3-hydroxy-2-[(E,3S)-3-hydroxyoct-1-enyl]-5-oxocyclopentyl]hept-5-enoic acid | |
---|---|---|
Source | PubChem | |
URL | https://pubchem.ncbi.nlm.nih.gov | |
Description | Data deposited in or computed by PubChem | |
InChI |
InChI=1S/C20H32O5/c1-2-3-6-9-15(21)12-13-17-16(18(22)14-19(17)23)10-7-4-5-8-11-20(24)25/h4,7,12-13,15-17,19,21,23H,2-3,5-6,8-11,14H2,1H3,(H,24,25)/b7-4-,13-12+/t15-,16+,17+,19+/m0/s1/i5D2,8D2 | |
Source | PubChem | |
URL | https://pubchem.ncbi.nlm.nih.gov | |
Description | Data deposited in or computed by PubChem | |
InChI Key |
XEYBRNLFEZDVAW-YOLMOHOWSA-N | |
Source | PubChem | |
URL | https://pubchem.ncbi.nlm.nih.gov | |
Description | Data deposited in or computed by PubChem | |
Canonical SMILES |
CCCCCC(C=CC1C(CC(=O)C1CC=CCCCC(=O)O)O)O | |
Source | PubChem | |
URL | https://pubchem.ncbi.nlm.nih.gov | |
Description | Data deposited in or computed by PubChem | |
Isomeric SMILES |
[2H]C([2H])(CC(=O)O)C([2H])([2H])/C=C\C[C@@H]1[C@H]([C@@H](CC1=O)O)/C=C/[C@H](CCCCC)O | |
Source | PubChem | |
URL | https://pubchem.ncbi.nlm.nih.gov | |
Description | Data deposited in or computed by PubChem | |
Molecular Formula |
C20H32O5 | |
Source | PubChem | |
URL | https://pubchem.ncbi.nlm.nih.gov | |
Description | Data deposited in or computed by PubChem | |
DSSTOX Substance ID |
DTXSID401347287 | |
Record name | Prostaglandin E2-3,3,4,4-d4 | |
Source | EPA DSSTox | |
URL | https://comptox.epa.gov/dashboard/DTXSID401347287 | |
Description | DSSTox provides a high quality public chemistry resource for supporting improved predictive toxicology. | |
Molecular Weight |
356.5 g/mol | |
Source | PubChem | |
URL | https://pubchem.ncbi.nlm.nih.gov | |
Description | Data deposited in or computed by PubChem | |
CAS No. |
34210-10-1 | |
Record name | Prostaglandin E2-3,3,4,4-d4 | |
Source | EPA DSSTox | |
URL | https://comptox.epa.gov/dashboard/DTXSID401347287 | |
Description | DSSTox provides a high quality public chemistry resource for supporting improved predictive toxicology. | |
Retrosynthesis Analysis
AI-Powered Synthesis Planning: Our tool employs the Template_relevance Pistachio, Template_relevance Bkms_metabolic, Template_relevance Pistachio_ringbreaker, Template_relevance Reaxys, Template_relevance Reaxys_biocatalysis model, leveraging a vast database of chemical reactions to predict feasible synthetic routes.
One-Step Synthesis Focus: Specifically designed for one-step synthesis, it provides concise and direct routes for your target compounds, streamlining the synthesis process.
Accurate Predictions: Utilizing the extensive PISTACHIO, BKMS_METABOLIC, PISTACHIO_RINGBREAKER, REAXYS, REAXYS_BIOCATALYSIS database, our tool offers high-accuracy predictions, reflecting the latest in chemical research and data.
Strategy Settings
Precursor scoring | Relevance Heuristic |
---|---|
Min. plausibility | 0.01 |
Model | Template_relevance |
Template Set | Pistachio/Bkms_metabolic/Pistachio_ringbreaker/Reaxys/Reaxys_biocatalysis |
Top-N result to add to graph | 6 |
Feasible Synthetic Routes
Disclaimer and Information on In-Vitro Research Products
Please be aware that all articles and product information presented on BenchChem are intended solely for informational purposes. The products available for purchase on BenchChem are specifically designed for in-vitro studies, which are conducted outside of living organisms. In-vitro studies, derived from the Latin term "in glass," involve experiments performed in controlled laboratory settings using cells or tissues. It is important to note that these products are not categorized as medicines or drugs, and they have not received approval from the FDA for the prevention, treatment, or cure of any medical condition, ailment, or disease. We must emphasize that any form of bodily introduction of these products into humans or animals is strictly prohibited by law. It is essential to adhere to these guidelines to ensure compliance with legal and ethical standards in research and experimentation.