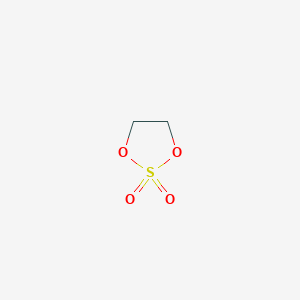
1,3,2-Dioxathiolane 2,2-dioxide
Overview
Description
Mechanism of Action
Target of Action
1,3,2-Dioxathiolane 2,2-dioxide (DTD) is an essential intermediate in organic synthesis and plays a vital role in improving the performance and service life of lithium-ion batteries . It primarily targets the solvation structure of Li-ion and the Solid Electrolyte Interphase (SEI) component .
Mode of Action
DTD interacts with its targets by changing the Li-ion solvation structure and optimizing the SEI component . This interaction decreases the energy barrier for Li deposition and reduces the generation of "dead Li" .
Biochemical Pathways
The primary biochemical pathway affected by DTD involves the synthesis process of DTD itself, which releases a large amount of heat, leading to its hydrolysis . This process plays a crucial role in the performance and service life of lithium-ion batteries .
Pharmacokinetics
It’s known that a large amount of heat is released during the synthesis process of dtd, leading to its hydrolysis . This could potentially impact the bioavailability of DTD.
Result of Action
The action of DTD results in a change in the deposition morphology of Li and effectively suppresses the growth of Li dendrites . This leads to an improvement in the performance and service life of lithium-ion batteries .
Action Environment
The action, efficacy, and stability of DTD are influenced by various environmental factors such as temperature, catalyst concentration, residence time, and the ratio of the two-phase flow rates . For instance, optimal conditions for the synthesis of DTD in microreactors have been determined to be a temperature of 14.73 °C, a catalyst concentration of 0.5 g L−1, a flow rate ratio between the continuous phase and the dispersed phase of 0.6, a total flow rate of 2 mL min−1, and a residence time of 117.75 s .
Biochemical Analysis
Biochemical Properties
It is known that it plays a vital role in the synthesis process of lithium-ion batteries . The exact enzymes, proteins, and other biomolecules it interacts with are yet to be identified.
Cellular Effects
It is known that a large amount of heat is released during its synthesis, leading to its hydrolysis . This could potentially influence cell function, including impacts on cell signaling pathways, gene expression, and cellular metabolism.
Molecular Mechanism
The molecular mechanism of 1,3,2-Dioxathiolane 2,2-dioxide involves its reduction prior to propylene carbonate (PC) and shows a strong reduction tendency accompanied by ring-opening . In its anionic form, this compound participates in the Li solvation sheath through a solvent–additive exchange reaction to promote the desolvation of PC .
Temporal Effects in Laboratory Settings
In laboratory settings, the synthesis of this compound has been optimized to achieve a continuous reaction yield of 92.22% . The synthesis kinetic data of this compound have been measured, providing insights into its stability, degradation, and long-term effects on cellular function .
Preparation Methods
1,3,2-Dioxathiolane 2,2-dioxide can be synthesized through various methods. One common synthetic route involves the reaction of ethylene glycol with thionyl chloride, using a heterogeneous catalyst such as ruthenium (III) chloride and various oxidants . The product is characterized by techniques such as 1H NMR and IR spectra .
Another method involves a two-step procedure where a 1,2-diol reacts with thionyl chloride to form a cyclic sulfite, which is then oxidized to the corresponding cyclic sulfate . Industrial production methods often utilize continuous flow microreaction technology, which offers advantages such as efficient dispersion and mixing of fluids at the micrometer scale. Optimal process conditions include a temperature of 14.73°C, a catalyst concentration of 0.5 g/L, and a total flow rate of 2 mL/min, resulting in a continuous reaction yield of 92.22% .
Chemical Reactions Analysis
1,3,2-Dioxathiolane 2,2-dioxide undergoes various chemical reactions, including oxidation, reduction, and substitution. Common reagents and conditions used in these reactions include thionyl chloride for the initial formation of cyclic sulfites and various oxidants for the subsequent oxidation to cyclic sulfates . Major products formed from these reactions include cyclic sulfates and other related compounds .
Scientific Research Applications
1,3,2-Dioxathiolane 2,2-dioxide has numerous scientific research applications. In chemistry, it is used as an intermediate in organic synthesis . In the field of energy storage, it plays a crucial role in improving the performance and service life of lithium-ion batteries by optimizing the solid-electrolyte interphase (SEI) component, which decreases the energy barrier for lithium deposition and reduces the generation of "dead lithium" . Additionally, it is used in the study of biomolecule-ligand complexes, free energy calculations, structure-based drug design, and refinement of x-ray crystal complexes .
Comparison with Similar Compounds
1,3,2-Dioxathiolane 2,2-dioxide can be compared with other similar compounds such as 1,3-propanesultone, 1,4-butane sultone, and ethylene sulfite . These compounds share similar structural features and applications in battery technology. this compound is unique in its ability to optimize the SEI component and improve the performance and lifespan of lithium-ion batteries .
Similar Compounds::- 1,3-Propanesultone
- 1,4-Butane sultone
- Ethylene sulfite
Properties
IUPAC Name |
1,3,2-dioxathiolane 2,2-dioxide | |
---|---|---|
Source | PubChem | |
URL | https://pubchem.ncbi.nlm.nih.gov | |
Description | Data deposited in or computed by PubChem | |
InChI |
InChI=1S/C2H4O4S/c3-7(4)5-1-2-6-7/h1-2H2 | |
Source | PubChem | |
URL | https://pubchem.ncbi.nlm.nih.gov | |
Description | Data deposited in or computed by PubChem | |
InChI Key |
ZPFAVCIQZKRBGF-UHFFFAOYSA-N | |
Source | PubChem | |
URL | https://pubchem.ncbi.nlm.nih.gov | |
Description | Data deposited in or computed by PubChem | |
Canonical SMILES |
C1COS(=O)(=O)O1 | |
Source | PubChem | |
URL | https://pubchem.ncbi.nlm.nih.gov | |
Description | Data deposited in or computed by PubChem | |
Molecular Formula |
C2H4O4S | |
Source | PubChem | |
URL | https://pubchem.ncbi.nlm.nih.gov | |
Description | Data deposited in or computed by PubChem | |
DSSTOX Substance ID |
DTXSID3020598 | |
Record name | Ethylene glycol, cyclic sulfate | |
Source | EPA DSSTox | |
URL | https://comptox.epa.gov/dashboard/DTXSID3020598 | |
Description | DSSTox provides a high quality public chemistry resource for supporting improved predictive toxicology. | |
Molecular Weight |
124.12 g/mol | |
Source | PubChem | |
URL | https://pubchem.ncbi.nlm.nih.gov | |
Description | Data deposited in or computed by PubChem | |
CAS No. |
1072-53-3 | |
Record name | 1,3,2-Dioxathiolane, 2,2-dioxide | |
Source | CAS Common Chemistry | |
URL | https://commonchemistry.cas.org/detail?cas_rn=1072-53-3 | |
Description | CAS Common Chemistry is an open community resource for accessing chemical information. Nearly 500,000 chemical substances from CAS REGISTRY cover areas of community interest, including common and frequently regulated chemicals, and those relevant to high school and undergraduate chemistry classes. This chemical information, curated by our expert scientists, is provided in alignment with our mission as a division of the American Chemical Society. | |
Explanation | The data from CAS Common Chemistry is provided under a CC-BY-NC 4.0 license, unless otherwise stated. | |
Record name | 1,3,2-Dioxathiolane, 2,2-dioxide | |
Source | ChemIDplus | |
URL | https://pubchem.ncbi.nlm.nih.gov/substance/?source=chemidplus&sourceid=0001072533 | |
Description | ChemIDplus is a free, web search system that provides access to the structure and nomenclature authority files used for the identification of chemical substances cited in National Library of Medicine (NLM) databases, including the TOXNET system. | |
Record name | Ethylene glycol, cyclic sulfate | |
Source | EPA DSSTox | |
URL | https://comptox.epa.gov/dashboard/DTXSID3020598 | |
Description | DSSTox provides a high quality public chemistry resource for supporting improved predictive toxicology. | |
Record name | 1,3,2-dioxathiolane 2,2-dioxide | |
Source | European Chemicals Agency (ECHA) | |
URL | https://echa.europa.eu/substance-information/-/substanceinfo/100.122.762 | |
Description | The European Chemicals Agency (ECHA) is an agency of the European Union which is the driving force among regulatory authorities in implementing the EU's groundbreaking chemicals legislation for the benefit of human health and the environment as well as for innovation and competitiveness. | |
Explanation | Use of the information, documents and data from the ECHA website is subject to the terms and conditions of this Legal Notice, and subject to other binding limitations provided for under applicable law, the information, documents and data made available on the ECHA website may be reproduced, distributed and/or used, totally or in part, for non-commercial purposes provided that ECHA is acknowledged as the source: "Source: European Chemicals Agency, http://echa.europa.eu/". Such acknowledgement must be included in each copy of the material. ECHA permits and encourages organisations and individuals to create links to the ECHA website under the following cumulative conditions: Links can only be made to webpages that provide a link to the Legal Notice page. | |
Retrosynthesis Analysis
AI-Powered Synthesis Planning: Our tool employs the Template_relevance Pistachio, Template_relevance Bkms_metabolic, Template_relevance Pistachio_ringbreaker, Template_relevance Reaxys, Template_relevance Reaxys_biocatalysis model, leveraging a vast database of chemical reactions to predict feasible synthetic routes.
One-Step Synthesis Focus: Specifically designed for one-step synthesis, it provides concise and direct routes for your target compounds, streamlining the synthesis process.
Accurate Predictions: Utilizing the extensive PISTACHIO, BKMS_METABOLIC, PISTACHIO_RINGBREAKER, REAXYS, REAXYS_BIOCATALYSIS database, our tool offers high-accuracy predictions, reflecting the latest in chemical research and data.
Strategy Settings
Precursor scoring | Relevance Heuristic |
---|---|
Min. plausibility | 0.01 |
Model | Template_relevance |
Template Set | Pistachio/Bkms_metabolic/Pistachio_ringbreaker/Reaxys/Reaxys_biocatalysis |
Top-N result to add to graph | 6 |
Feasible Synthetic Routes
Disclaimer and Information on In-Vitro Research Products
Please be aware that all articles and product information presented on BenchChem are intended solely for informational purposes. The products available for purchase on BenchChem are specifically designed for in-vitro studies, which are conducted outside of living organisms. In-vitro studies, derived from the Latin term "in glass," involve experiments performed in controlled laboratory settings using cells or tissues. It is important to note that these products are not categorized as medicines or drugs, and they have not received approval from the FDA for the prevention, treatment, or cure of any medical condition, ailment, or disease. We must emphasize that any form of bodily introduction of these products into humans or animals is strictly prohibited by law. It is essential to adhere to these guidelines to ensure compliance with legal and ethical standards in research and experimentation.