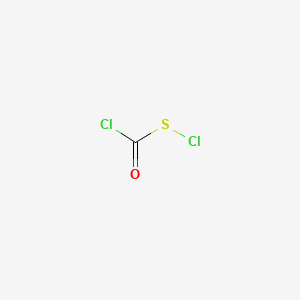
Chlorocarbonylsulfenyl chloride
Overview
Description
Chlorocarbonylsulfenyl chloride is a chemical compound with the formula ClCOSCl. It is a yellow liquid that fumes in moist air . It has been used in the preparation of various sulfur heterocycles and other compounds .
Synthesis Analysis
The synthesis of this compound has been described in various studies. For instance, it has been used in the preparation of 5-(1,2,3,4-tetra-O-acetyl-alpha-D-xylopyranos-5 S - C -yl)-1,3,4-oxathiazol-2-one . Another study describes the preparation of [18O]‐this compound .
Molecular Structure Analysis
The molecular structure of this compound has been studied using various techniques. A study published in the Journal of Labelled Compounds and Radiopharmaceuticals describes the preparation of [18O]‐this compound . Another study found that gaseous this compound has a major conformer with the chlorine atoms anti to each other .
Chemical Reactions Analysis
This compound has been used in various chemical reactions. For instance, it has been used in the preparation of 5-(1,2,3,4-tetra-O-acetyl-alpha-D-xylopyranos-5 S - C -yl)-1,3,4-oxathiazol-2-one . It has also been used in the preparation of fluorinated oxathialones .
Physical And Chemical Properties Analysis
This compound is a yellow liquid that fumes in moist air . It has a refractive index of 1.517 (lit.) and a boiling point of 98 °C (lit.). The density of this compound is 1.552 g/mL at 25 °C (lit.) .
Scientific Research Applications
Molecular Structure and Conformation
Chlorocarbonylsulfenyl chloride has been studied for its molecular structure and conformation. Electron diffraction investigations at 35°C revealed that the major conformer has chlorine atoms positioned anti to each other. This research provides fundamental insights into the molecular architecture of this compound, which is essential for understanding its reactivity and potential applications in various fields (Shen & Hagen, 1985).
Heterocyclization Reactions
In the realm of synthetic chemistry, this compound has been utilized in novel heterocyclization reactions. It reacts with 1-alkylidene-4-phenylthiosemicarbazones to yield 1,2,4-triazolines, and with 1-arylidene-4-phenylthiosemicarbazones to afford 1,2,4-dithiazolidines. This showcases its versatility in synthesizing heterocyclic compounds, which are important in pharmaceuticals and agrochemicals (Kabashima, Okawara, Yamasaki, & Furukawa, 1991).
Isotopic Enrichment
This compound has been used in the preparation of isotopically enriched compounds, such as [18O]-chlorocarbonylsulfenyl chloride. The process involves a series of steps starting with the [18O]-hydrolysis of 2-ethyl-1,3-dioxolane. This application is significant in the field of isotopic labeling, which is crucial for tracing chemical reactions and studying mechanism pathways (Mott, Eastep, Słomczyńska, & Bárány, 1984).
Synthesis of Chlorosulfenylated Carbonic Acid Derivatives
Research has been conducted on the synthesis of this compound and its imino analogs. The chemical behavior of these bifunctional compounds has been explored, particularly in synthesizing five-membered heterocycles containing sulfur, oxygen, and/or nitrogen. This application is important in the development of new chemical entities with potential industrial and medicinal applications (Zumach & Kuhle, 1970).
Coulomb Explosion Dynamics
The Coulomb explosion dynamics of this compound following strong field ionization have been studied, showcasing its use in advanced physical chemistry experiments. These studies help in understanding the molecular dissociation processes under extreme conditions, which is valuable for research in fields like mass spectrometry and nuclear chemistry (Cooper, Alavi, Li, Lee, & Suits, 2021).
Safety and Hazards
Chlorocarbonylsulfenyl chloride is classified as a combustible liquid and causes severe skin burns and eye damage . It is recommended to avoid breathing its dust/fume/gas/mist/vapors/spray and to wash thoroughly after handling. Protective gloves, clothing, and eye/face protection should be worn when handling this chemical .
Relevant Papers
Several papers have been published on this compound. For instance, a paper titled “Coulomb Explosion Dynamics of this compound” discusses the Coulomb explosion dynamics following strong field ionization of this compound . Another paper titled “Regioselective Cyclocondensations of this compound with Hydrazones: Effective Synthesis of a Class of Sulfur and Nitrogen Containing Heterocycles with ‐COS‐ Linkage” discusses the regioselective cyclocondensations of this compound with hydrazones .
Mechanism of Action
Target of Action
Chlorocarbonylsulfenyl chloride is a chemical compound with the formula CCl2OS It’s known that the compound interacts with various substances in the preparation of several other compounds .
Mode of Action
It’s known to be used in the synthesis of various compounds, indicating that it likely reacts with other molecules to form new chemical structures .
Biochemical Pathways
It has been used in the preparation of several compounds, including 5-(1,2,3,4-tetra-o-acetyl-alpha-d-xylopyranos-5s-c-yl)-1,3,4-oxathiazol-2-one, fluorinated oxathialones, polyfluoroalkylchlorothioformates, chlorocarbonylpolyfluoroalkylsulfenate esters, and chlorocarbonylhexafluoroisopropylidenimino sulfenate . This suggests that it may play a role in various chemical reactions and pathways.
Pharmacokinetics
As a chemical reagent, it’s primarily used in laboratory settings for the synthesis of other compounds .
Result of Action
Its use in the synthesis of various compounds suggests that it can facilitate significant chemical transformations .
Action Environment
The action of this compound can be influenced by environmental factors such as temperature and storage conditions. It’s recommended to store the compound in a cool, well-ventilated place . It’s also important to handle and open the container with care due to its corrosive nature .
Properties
IUPAC Name |
S-chloro chloromethanethioate | |
---|---|---|
Source | PubChem | |
URL | https://pubchem.ncbi.nlm.nih.gov | |
Description | Data deposited in or computed by PubChem | |
InChI |
InChI=1S/CCl2OS/c2-1(4)5-3 | |
Source | PubChem | |
URL | https://pubchem.ncbi.nlm.nih.gov | |
Description | Data deposited in or computed by PubChem | |
InChI Key |
MNOALXGAYUJNKX-UHFFFAOYSA-N | |
Source | PubChem | |
URL | https://pubchem.ncbi.nlm.nih.gov | |
Description | Data deposited in or computed by PubChem | |
Canonical SMILES |
C(=O)(SCl)Cl | |
Source | PubChem | |
URL | https://pubchem.ncbi.nlm.nih.gov | |
Description | Data deposited in or computed by PubChem | |
Molecular Formula |
CCl2OS | |
Source | PubChem | |
URL | https://pubchem.ncbi.nlm.nih.gov | |
Description | Data deposited in or computed by PubChem | |
DSSTOX Substance ID |
DTXSID10181970 | |
Record name | (Chlorothio)formyl chloride | |
Source | EPA DSSTox | |
URL | https://comptox.epa.gov/dashboard/DTXSID10181970 | |
Description | DSSTox provides a high quality public chemistry resource for supporting improved predictive toxicology. | |
Molecular Weight |
130.98 g/mol | |
Source | PubChem | |
URL | https://pubchem.ncbi.nlm.nih.gov | |
Description | Data deposited in or computed by PubChem | |
CAS No. |
2757-23-5 | |
Record name | (Chlorocarbonyl)sulfenyl chloride | |
Source | CAS Common Chemistry | |
URL | https://commonchemistry.cas.org/detail?cas_rn=2757-23-5 | |
Description | CAS Common Chemistry is an open community resource for accessing chemical information. Nearly 500,000 chemical substances from CAS REGISTRY cover areas of community interest, including common and frequently regulated chemicals, and those relevant to high school and undergraduate chemistry classes. This chemical information, curated by our expert scientists, is provided in alignment with our mission as a division of the American Chemical Society. | |
Explanation | The data from CAS Common Chemistry is provided under a CC-BY-NC 4.0 license, unless otherwise stated. | |
Record name | (Chlorothio)formyl chloride | |
Source | ChemIDplus | |
URL | https://pubchem.ncbi.nlm.nih.gov/substance/?source=chemidplus&sourceid=0002757235 | |
Description | ChemIDplus is a free, web search system that provides access to the structure and nomenclature authority files used for the identification of chemical substances cited in National Library of Medicine (NLM) databases, including the TOXNET system. | |
Record name | (Chlorothio)formyl chloride | |
Source | EPA DSSTox | |
URL | https://comptox.epa.gov/dashboard/DTXSID10181970 | |
Description | DSSTox provides a high quality public chemistry resource for supporting improved predictive toxicology. | |
Record name | (chlorothio)formyl chloride | |
Source | European Chemicals Agency (ECHA) | |
URL | https://echa.europa.eu/substance-information/-/substanceinfo/100.018.560 | |
Description | The European Chemicals Agency (ECHA) is an agency of the European Union which is the driving force among regulatory authorities in implementing the EU's groundbreaking chemicals legislation for the benefit of human health and the environment as well as for innovation and competitiveness. | |
Explanation | Use of the information, documents and data from the ECHA website is subject to the terms and conditions of this Legal Notice, and subject to other binding limitations provided for under applicable law, the information, documents and data made available on the ECHA website may be reproduced, distributed and/or used, totally or in part, for non-commercial purposes provided that ECHA is acknowledged as the source: "Source: European Chemicals Agency, http://echa.europa.eu/". Such acknowledgement must be included in each copy of the material. ECHA permits and encourages organisations and individuals to create links to the ECHA website under the following cumulative conditions: Links can only be made to webpages that provide a link to the Legal Notice page. | |
Synthesis routes and methods
Procedure details
Retrosynthesis Analysis
AI-Powered Synthesis Planning: Our tool employs the Template_relevance Pistachio, Template_relevance Bkms_metabolic, Template_relevance Pistachio_ringbreaker, Template_relevance Reaxys, Template_relevance Reaxys_biocatalysis model, leveraging a vast database of chemical reactions to predict feasible synthetic routes.
One-Step Synthesis Focus: Specifically designed for one-step synthesis, it provides concise and direct routes for your target compounds, streamlining the synthesis process.
Accurate Predictions: Utilizing the extensive PISTACHIO, BKMS_METABOLIC, PISTACHIO_RINGBREAKER, REAXYS, REAXYS_BIOCATALYSIS database, our tool offers high-accuracy predictions, reflecting the latest in chemical research and data.
Strategy Settings
Precursor scoring | Relevance Heuristic |
---|---|
Min. plausibility | 0.01 |
Model | Template_relevance |
Template Set | Pistachio/Bkms_metabolic/Pistachio_ringbreaker/Reaxys/Reaxys_biocatalysis |
Top-N result to add to graph | 6 |
Feasible Synthetic Routes
Disclaimer and Information on In-Vitro Research Products
Please be aware that all articles and product information presented on BenchChem are intended solely for informational purposes. The products available for purchase on BenchChem are specifically designed for in-vitro studies, which are conducted outside of living organisms. In-vitro studies, derived from the Latin term "in glass," involve experiments performed in controlled laboratory settings using cells or tissues. It is important to note that these products are not categorized as medicines or drugs, and they have not received approval from the FDA for the prevention, treatment, or cure of any medical condition, ailment, or disease. We must emphasize that any form of bodily introduction of these products into humans or animals is strictly prohibited by law. It is essential to adhere to these guidelines to ensure compliance with legal and ethical standards in research and experimentation.