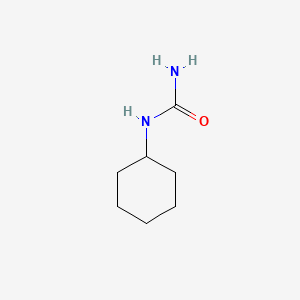
Cyclohexylurea
Overview
Description
Synthesis Analysis
The classical approach for the preparation of urea derivatives, such as Cyclohexylurea, involves reagents such as phosgene or its derivative, triphosgene. Urea synthesis typically proceeds through an isocyanate intermediate, which upon reaction with a second amine forms the urea derivatives .Molecular Structure Analysis
The molecular weight of this compound is 142.20 g/mol . The IUPAC name for this compound is this compound . The InChI representation of the molecule is InChI=1S/C7H14N2O/c8-7(10)9-6-4-2-1-3-5-6/h6H,1-5H2,(H3,8,9,10) .Physical And Chemical Properties Analysis
This compound has a molecular weight of 142.20 g/mol . It has a hydrogen bond donor count of 2 and a hydrogen bond acceptor count of 1 . The topological polar surface area of this compound is 55.1 Ų . The density of this compound is 1.1±0.1 g/cm³ . The boiling point is 240.3±13.0 °C at 760 mmHg .Scientific Research Applications
Neuropeptide Y (NPY) Y5 Receptor Antagonists : Cyclohexylurea derivatives have been studied for their role as potent NPY Y5 receptor antagonists. For instance, this compound 21c has shown promising results in reducing food intake, body weight gain, and adipose mass in diet-induced obese rats, indicating its potential application in anti-obesity treatments (Li et al., 2008).
Cancer Research : Studies have examined the absorption, distribution, excretion, and biotransformation of 1-(2-chloroethyl)-3-cyclohexyl-1-nitrosourea, a compound known for its effectiveness against mouse leukemia L1210. This research is vital for understanding the pharmacokinetics and mechanism of action of this compound derivatives in cancer therapy (Oliverio et al., 1970).
Biochemical Studies : Cycloheximide, a related compound, has been used to study the entrance of messenger RNA into membrane-bound and free polyribosomes, offering insights into the complex mechanisms of protein synthesis at the cellular level (Rosbash, 1972).
Chemosensors : Research into fluorescent cyclohexyl-based chemosensors has been conducted, with studies showing their effectiveness in selectively sensing specific compounds in mixed environments. This has implications for environmental monitoring and chemical detection (Costero et al., 2009).
Anti-Tuberculosis and Anti-Cancer Agents : Novel acyl thiourea derivatives synthesized from cyclohexanecarbonyl isothiocyanate have shown good anti-oxidant and anti-haemolytic activities. These compounds have been screened against enzymes targeting tuberculosis and cancer, highlighting their potential therapeutic applications (Haribabu et al., 2015).
Environmental Protection : Studies on cyclohexane and sec-butyl alcohol, which are used in the pharmaceutical and chemical industries, highlight the importance of environmentally sustainable separation and recovery processes. This research contributes to mitigating environmental risks and promotes sustainable development (Zhu et al., 2021).
- lohexyl isocyanate has revealed their potential as biomaterials for medical applications. These films have been evaluated for their chemical, physico-mechanical, and biological properties, indicating their suitability in various medical contexts (Król et al., 2018).
Molecular Interactions in Cancer Therapeutics : Studies on the binding of 1-(2-chloroethyl)-3-cyclohexyl-1-nitrosourea with nucleic acids and proteins provide insights into the dual capacity of the drug in modifying cellular proteins and nucleic acids, a factor important in understanding its carcinostatic activity (Cheng et al., 1972).
Synthesis of Novel Polyureas : Research on the synthesis of new polyureas derived from cyclohexylurazole has contributed to the development of materials with potential applications in various industrial sectors. The physical properties and structural characterization of these polyureas have been extensively studied (Mallakpour & Rostamizadeh, 2001).
Inhibition of Steroid-Induced Behavior : Cycloheximide has been used in studies exploring the inhibition of estrogen-induced sexual receptivity in animals, offering insights into the complex interactions between hormones and behavior (Quadagno & Ho, 1975).
DNA Replication and Protein Synthesis : Investigations into the interrelationship between nuclear DNA replication and cytoplasmic protein synthesis in Chlorella using cycloheximide provide a deeper understanding of cellular processes and the effects of specific inhibitors (Wanka et al., 1972).
Template-Controlled Synthesis : The template-controlled synthesis of cyclohexylhemicucurbit[8]uril, a molecule with a barrel-shaped cavity, highlights advances in organic synthesis and molecular design, with potential applications in material science and molecular recognition (Prigorchenko et al., 2015).
Cyclohexane Emission Removal : Studies on the removal of cyclohexane gaseous emissions using biotrickling filter systems demonstrate the potential for biological methods in environmental protection and industrial emission control (Salamanca et al., 2017).
- '-methylenedianiline (MDA), a carcinogen produced from the degradation of polyurethane in medical devices, has been significant for understanding the implications of medical device sterilization and ensuring patient safety (Shintani, 1991).
Protein Synthesis Inhibition : Cycloheximide derivatives have been developed to enhance the inhibition of translation elongation in protein synthesis studies. These derivatives offer more effective capture of ribosome conformations and improved potency in ribosome profiling experiments, making them valuable tools in molecular biology research (Koga et al., 2021).
Hydrodeoxygenation of Aliphatic Ketones : Cyclohexanone, used as a model substrate, has been evaluated in the hydrodeoxygenation process over alkali-treated catalysts. This research contributes to the development of efficient and environmentally sustainable methods for transforming ketones into useful alkanes (Kong et al., 2013).
Safety and Hazards
Future Directions
The Cyclohexylurea market is experiencing significant growth driven by multiple factors. Technological advancements are continuously improving this compound products, making them more efficient and versatile. This, coupled with increasing awareness among consumers about the benefits of this compound, is fueling demand across various industries .
Mechanism of Action
Biochemical Analysis
Biochemical Properties
Cyclohexylurea plays a significant role in various biochemical reactions. It interacts with several enzymes, proteins, and other biomolecules. For instance, this compound is known to inhibit certain proteases, which are enzymes that break down proteins. This inhibition occurs through the formation of a stable complex between this compound and the active site of the enzyme, preventing the enzyme from catalyzing its substrate. Additionally, this compound can interact with transport proteins, affecting the transport of other molecules across cell membranes .
Cellular Effects
This compound has been shown to influence various cellular processes. It can affect cell signaling pathways, gene expression, and cellular metabolism. For example, this compound can modulate the activity of signaling pathways such as the NF-κB pathway, which plays a crucial role in inflammation and immune responses. By inhibiting specific enzymes, this compound can alter gene expression patterns, leading to changes in cellular functions. Furthermore, this compound can impact cellular metabolism by affecting the activity of metabolic enzymes .
Molecular Mechanism
The molecular mechanism of this compound involves its interaction with various biomolecules. This compound can bind to the active sites of enzymes, leading to enzyme inhibition or activation. For instance, this compound can inhibit proteases by forming a stable complex with the enzyme, preventing it from catalyzing its substrate. Additionally, this compound can influence gene expression by interacting with transcription factors and other regulatory proteins. These interactions can lead to changes in the expression of specific genes, ultimately affecting cellular functions .
Temporal Effects in Laboratory Settings
In laboratory settings, the effects of this compound can change over time. This compound is a stable compound that maintains its structural integrity at high temperatures. Its stability can be affected by factors such as pH and the presence of other chemicals. Over time, this compound can undergo degradation, leading to changes in its effects on cellular functions. Long-term studies have shown that this compound can have lasting effects on cellular processes, including alterations in gene expression and enzyme activity .
Dosage Effects in Animal Models
The effects of this compound can vary with different dosages in animal models. At low doses, this compound can have beneficial effects, such as inhibiting specific enzymes and modulating cellular functions. At high doses, this compound can exhibit toxic effects, including cell death and tissue damage. Studies have shown that there is a threshold dose above which the adverse effects of this compound become significant. It is crucial to determine the optimal dosage to maximize the beneficial effects while minimizing the toxic effects .
Metabolic Pathways
This compound is involved in various metabolic pathways. It can interact with enzymes and cofactors, affecting metabolic flux and metabolite levels. For example, this compound can inhibit enzymes involved in the urea cycle, leading to changes in the levels of urea and other metabolites. Additionally, this compound can influence the activity of enzymes involved in amino acid metabolism, affecting the synthesis and degradation of amino acids .
Transport and Distribution
This compound is transported and distributed within cells and tissues through various mechanisms. It can interact with transport proteins, facilitating its movement across cell membranes. Once inside the cell, this compound can be distributed to different cellular compartments, including the cytoplasm and organelles. The distribution of this compound can be influenced by factors such as pH, temperature, and the presence of other molecules .
Subcellular Localization
The subcellular localization of this compound can affect its activity and function. This compound can be directed to specific compartments or organelles through targeting signals or post-translational modifications. For instance, this compound can be localized to the mitochondria, where it can influence mitochondrial functions such as energy production and apoptosis. Additionally, this compound can be found in the nucleus, where it can interact with transcription factors and other regulatory proteins, affecting gene expression .
Properties
IUPAC Name |
cyclohexylurea | |
---|---|---|
Source | PubChem | |
URL | https://pubchem.ncbi.nlm.nih.gov | |
Description | Data deposited in or computed by PubChem | |
InChI |
InChI=1S/C7H14N2O/c8-7(10)9-6-4-2-1-3-5-6/h6H,1-5H2,(H3,8,9,10) | |
Source | PubChem | |
URL | https://pubchem.ncbi.nlm.nih.gov | |
Description | Data deposited in or computed by PubChem | |
InChI Key |
WUESWDIHTKHGQA-UHFFFAOYSA-N | |
Source | PubChem | |
URL | https://pubchem.ncbi.nlm.nih.gov | |
Description | Data deposited in or computed by PubChem | |
Canonical SMILES |
C1CCC(CC1)NC(=O)N | |
Source | PubChem | |
URL | https://pubchem.ncbi.nlm.nih.gov | |
Description | Data deposited in or computed by PubChem | |
Molecular Formula |
C7H14N2O | |
Source | PubChem | |
URL | https://pubchem.ncbi.nlm.nih.gov | |
Description | Data deposited in or computed by PubChem | |
DSSTOX Substance ID |
DTXSID20220102 | |
Record name | Urea, cyclohexyl- | |
Source | EPA DSSTox | |
URL | https://comptox.epa.gov/dashboard/DTXSID20220102 | |
Description | DSSTox provides a high quality public chemistry resource for supporting improved predictive toxicology. | |
Molecular Weight |
142.20 g/mol | |
Source | PubChem | |
URL | https://pubchem.ncbi.nlm.nih.gov | |
Description | Data deposited in or computed by PubChem | |
CAS No. |
698-90-8 | |
Record name | Cyclohexylurea | |
Source | CAS Common Chemistry | |
URL | https://commonchemistry.cas.org/detail?cas_rn=698-90-8 | |
Description | CAS Common Chemistry is an open community resource for accessing chemical information. Nearly 500,000 chemical substances from CAS REGISTRY cover areas of community interest, including common and frequently regulated chemicals, and those relevant to high school and undergraduate chemistry classes. This chemical information, curated by our expert scientists, is provided in alignment with our mission as a division of the American Chemical Society. | |
Explanation | The data from CAS Common Chemistry is provided under a CC-BY-NC 4.0 license, unless otherwise stated. | |
Record name | Cyclohexylurea | |
Source | ChemIDplus | |
URL | https://pubchem.ncbi.nlm.nih.gov/substance/?source=chemidplus&sourceid=0000698908 | |
Description | ChemIDplus is a free, web search system that provides access to the structure and nomenclature authority files used for the identification of chemical substances cited in National Library of Medicine (NLM) databases, including the TOXNET system. | |
Record name | Cyclohexylurea | |
Source | DTP/NCI | |
URL | https://dtp.cancer.gov/dtpstandard/servlet/dwindex?searchtype=NSC&outputformat=html&searchlist=27454 | |
Description | The NCI Development Therapeutics Program (DTP) provides services and resources to the academic and private-sector research communities worldwide to facilitate the discovery and development of new cancer therapeutic agents. | |
Explanation | Unless otherwise indicated, all text within NCI products is free of copyright and may be reused without our permission. Credit the National Cancer Institute as the source. | |
Record name | Cyclohexylurea | |
Source | DTP/NCI | |
URL | https://dtp.cancer.gov/dtpstandard/servlet/dwindex?searchtype=NSC&outputformat=html&searchlist=23790 | |
Description | The NCI Development Therapeutics Program (DTP) provides services and resources to the academic and private-sector research communities worldwide to facilitate the discovery and development of new cancer therapeutic agents. | |
Explanation | Unless otherwise indicated, all text within NCI products is free of copyright and may be reused without our permission. Credit the National Cancer Institute as the source. | |
Record name | Urea, cyclohexyl- | |
Source | EPA DSSTox | |
URL | https://comptox.epa.gov/dashboard/DTXSID20220102 | |
Description | DSSTox provides a high quality public chemistry resource for supporting improved predictive toxicology. | |
Record name | Cyclohexylurea | |
Source | European Chemicals Agency (ECHA) | |
URL | https://echa.europa.eu/substance-information/-/substanceinfo/100.010.749 | |
Description | The European Chemicals Agency (ECHA) is an agency of the European Union which is the driving force among regulatory authorities in implementing the EU's groundbreaking chemicals legislation for the benefit of human health and the environment as well as for innovation and competitiveness. | |
Explanation | Use of the information, documents and data from the ECHA website is subject to the terms and conditions of this Legal Notice, and subject to other binding limitations provided for under applicable law, the information, documents and data made available on the ECHA website may be reproduced, distributed and/or used, totally or in part, for non-commercial purposes provided that ECHA is acknowledged as the source: "Source: European Chemicals Agency, http://echa.europa.eu/". Such acknowledgement must be included in each copy of the material. ECHA permits and encourages organisations and individuals to create links to the ECHA website under the following cumulative conditions: Links can only be made to webpages that provide a link to the Legal Notice page. | |
Record name | CYCLOHEXYLUREA | |
Source | FDA Global Substance Registration System (GSRS) | |
URL | https://gsrs.ncats.nih.gov/ginas/app/beta/substances/EYE4CFF4RV | |
Description | The FDA Global Substance Registration System (GSRS) enables the efficient and accurate exchange of information on what substances are in regulated products. Instead of relying on names, which vary across regulatory domains, countries, and regions, the GSRS knowledge base makes it possible for substances to be defined by standardized, scientific descriptions. | |
Explanation | Unless otherwise noted, the contents of the FDA website (www.fda.gov), both text and graphics, are not copyrighted. They are in the public domain and may be republished, reprinted and otherwise used freely by anyone without the need to obtain permission from FDA. Credit to the U.S. Food and Drug Administration as the source is appreciated but not required. | |
Synthesis routes and methods I
Procedure details
Synthesis routes and methods II
Procedure details
Synthesis routes and methods III
Procedure details
Synthesis routes and methods IV
Procedure details
Retrosynthesis Analysis
AI-Powered Synthesis Planning: Our tool employs the Template_relevance Pistachio, Template_relevance Bkms_metabolic, Template_relevance Pistachio_ringbreaker, Template_relevance Reaxys, Template_relevance Reaxys_biocatalysis model, leveraging a vast database of chemical reactions to predict feasible synthetic routes.
One-Step Synthesis Focus: Specifically designed for one-step synthesis, it provides concise and direct routes for your target compounds, streamlining the synthesis process.
Accurate Predictions: Utilizing the extensive PISTACHIO, BKMS_METABOLIC, PISTACHIO_RINGBREAKER, REAXYS, REAXYS_BIOCATALYSIS database, our tool offers high-accuracy predictions, reflecting the latest in chemical research and data.
Strategy Settings
Precursor scoring | Relevance Heuristic |
---|---|
Min. plausibility | 0.01 |
Model | Template_relevance |
Template Set | Pistachio/Bkms_metabolic/Pistachio_ringbreaker/Reaxys/Reaxys_biocatalysis |
Top-N result to add to graph | 6 |
Feasible Synthetic Routes
Disclaimer and Information on In-Vitro Research Products
Please be aware that all articles and product information presented on BenchChem are intended solely for informational purposes. The products available for purchase on BenchChem are specifically designed for in-vitro studies, which are conducted outside of living organisms. In-vitro studies, derived from the Latin term "in glass," involve experiments performed in controlled laboratory settings using cells or tissues. It is important to note that these products are not categorized as medicines or drugs, and they have not received approval from the FDA for the prevention, treatment, or cure of any medical condition, ailment, or disease. We must emphasize that any form of bodily introduction of these products into humans or animals is strictly prohibited by law. It is essential to adhere to these guidelines to ensure compliance with legal and ethical standards in research and experimentation.