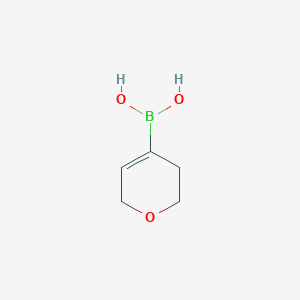
(3,6-Dihydro-2H-pyran-4-YL)boronic acid
Overview
Description
(3,6-Dihydro-2H-pyran-4-YL)boronic acid (chemical formula: C₅H₉BO₃, CAS: 1002127-60-7) is a cyclic organoboron compound featuring a partially unsaturated dihydropyran ring. This boronic acid derivative is synthesized via palladium-catalyzed cross-coupling reactions or through functionalization of preformed dihydropyran intermediates . Its pinacol ester derivatives, such as 2-(3,6-Dihydro-2H-pyran-4-yl)-4,4,5,5-tetramethyl-1,3,2-dioxaborolane, are widely used in Suzuki-Miyaura couplings to access complex heterocyclic scaffolds . The compound’s stability and reactivity stem from its boronic acid moiety, which enables reversible interactions with diols and amines, making it valuable in drug discovery and materials science .
Preparation Methods
Hydroboration of 3,6-Dihydro-2H-pyran
One classical method involves the hydroboration of 3,6-dihydro-2H-pyran using borane reagents. The reaction proceeds under an inert atmosphere (e.g., nitrogen or argon) at low temperatures to avoid decomposition of sensitive borane intermediates. After hydroboration, oxidation yields the boronic acid product.
- Reaction Conditions:
- Inert atmosphere (N2 or Ar)
- Low temperature (typically 0 to 25 °C)
- Borane reagents such as BH3·THF complex
- Advantages: Straightforward, direct conversion from pyran to boronic acid
- Limitations: Requires careful control of moisture and temperature to prevent side reactions and decomposition
Palladium-Catalyzed Borylation of Halogenated Pyran Intermediates
An efficient and scalable method involves the preparation of halogenated pyran intermediates (e.g., 4-bromo-3,6-dihydro-2H-pyran) followed by palladium-catalyzed borylation using bis(pinacolato)diboron.
- Step 1: Preparation of 4-bromo-3,6-dihydro-2H-pyran
- Starting from 4-tetrahydropyranone, condensation with hydrazine hydrate forms a hydrazone intermediate.
- Treatment of this hydrazone with copper(II) bromide-triethylamine complex and DBU (1,8-diazabicyclo[5.4.0]undec-7-ene) produces the 4-bromo derivative.
Step 2: Palladium-catalyzed borylation
- Using 1% mol Pd catalyst and bis(pinacolato)diboron under mild conditions produces the boronic acid pinacol ester.
- The pinacol ester can be hydrolyzed to yield the free boronic acid.
-
- Pd catalyst (e.g., PdCl2(dppf))
- Potassium acetate as base
- Solvent: 1,4-dioxane
- Temperature: ~80 °C
- Inert atmosphere (argon)
- Yield: Approximately 66% for the borylation step; overall process is efficient and scalable
- Advantages: One-pot synthesis, avoids laborious purification steps, suitable for industrial scale
- References: Feng Xue et al., 2015; chemical patent WO2008/153858
One-Pot Synthesis of 3,6-Dihydro-2H-pyran-4-boronic acid Pinacol Ester
An improved one-pot synthetic process has been developed to simplify the preparation, starting from 4-tetrahydropyranone and hydrazine hydrate without the need for molecular sieves. This method avoids the formation of azines and allows for relatively pure hydrazone formation, which is then converted to the boronic acid pinacol ester via copper(II)-mediated halogenation and palladium-catalyzed borylation.
- Key Features:
- No molecular sieves required
- Simplified reaction steps
- Good yield and purity
- Significance: More economical and effective for both laboratory and industrial production
Synthesis via Trifluoromethanesulfonate Intermediate
Another method involves the synthesis of 3,6-dihydro-2H-pyran-4-boronic acid pinacol ester by palladium-catalyzed borylation of 3,6-dihydro-2H-pyran-4-yl trifluoromethanesulfonate.
- Reaction Conditions:
- PdCl2(dppf) catalyst (1,1'-bis(diphenylphosphino)ferrocene palladium(II) dichloride)
- Potassium acetate as base
- Solvent: 1,4-dioxane
- Temperature: 80 °C, overnight
- Yield: 81% isolated yield of pinacol ester
- Purification: Column chromatography (10% hexane in dichloromethane)
- Advantages: High yield, straightforward purification
- Reference: Schering Corporation patent WO2008/153858
Method | Starting Material | Key Reagents/Conditions | Yield (%) | Advantages | Limitations |
---|---|---|---|---|---|
Hydroboration of 3,6-dihydro-2H-pyran | 3,6-Dihydro-2H-pyran | Borane reagents, inert atmosphere, low temp | Moderate | Direct synthesis | Sensitive to moisture, temperature |
Pd-Catalyzed Borylation of 4-bromo-3,6-dihydro-2H-pyran | 4-Tetrahydropyranone → hydrazone → 4-bromo derivative | Pd catalyst, bis(pinacolato)diboron, KAc, dioxane, 80 °C | ~66 | Scalable, efficient, one-pot | Requires preparation of halogenated intermediate |
One-pot synthesis via hydrazone and copper(II) bromide | 4-Tetrahydropyranone + hydrazine hydrate | Copper(II) bromide, Pd catalyst, DBU | Good | Simplified, avoids molecular sieves | Multi-step but streamlined |
Pd-catalyzed borylation of trifluoromethanesulfonate ester | 3,6-Dihydro-2H-pyran-4-yl triflate | PdCl2(dppf), potassium acetate, 1,4-dioxane, 80 °C | 81 | High yield, easy purification | Requires triflate intermediate |
- The palladium-catalyzed borylation methods are currently the most efficient and practical for laboratory and industrial scale synthesis due to their high yields, mild reaction conditions, and scalability.
- The one-pot hydrazone-based synthesis improves safety and simplicity by circumventing the need for molecular sieves and complicated purification steps.
- Hydroboration remains a classical approach but is less favored industrially due to sensitivity to moisture and handling difficulties of borane reagents.
- The use of pinacol esters as intermediates is common, as they are more stable and easier to handle than free boronic acids, which are prone to oxidation and polymerization.
- Reaction conditions such as inert atmosphere, temperature control, and choice of base (potassium acetate) are critical to maximize yield and purity.
The preparation of (3,6-Dihydro-2H-pyran-4-yl)boronic acid is well-established through multiple synthetic routes, with palladium-catalyzed borylation of halogenated pyran derivatives currently representing the most efficient and scalable approach. Advances in one-pot synthesis methods have further improved the practicality and economics of production. These methods provide reliable access to this valuable boronic acid derivative for use in organic synthesis and pharmaceutical applications.
Chemical Reactions Analysis
Types of Reactions
(3,6-Dihydro-2H-pyran-4-YL)boronic acid undergoes several types of chemical reactions, including:
Oxidation: The boronic acid group can be oxidized to form borate esters or boronic anhydrides.
Reduction: Reduction reactions can convert the boronic acid to borane derivatives.
Substitution: The compound can participate in nucleophilic substitution reactions, where the boronic acid group is replaced by other functional groups.
Common Reagents and Conditions
Oxidation: Common oxidizing agents include hydrogen peroxide and sodium perborate.
Reduction: Reducing agents such as lithium aluminum hydride or sodium borohydride are typically used.
Substitution: Nucleophiles like amines or alcohols can be used under mild conditions to achieve substitution reactions.
Major Products Formed
Oxidation: Borate esters and boronic anhydrides.
Reduction: Borane derivatives.
Substitution: Various substituted pyran derivatives depending on the nucleophile used.
Scientific Research Applications
Synthetic Applications
-
Intermediate for Organic Synthesis:
- (3,6-Dihydro-2H-pyran-4-YL)boronic acid serves as an important intermediate in the synthesis of various organic compounds, including pharmaceuticals and agrochemicals. It is particularly useful in the preparation of complex molecules through cross-coupling reactions like Suzuki-Miyaura coupling, which involves the formation of carbon-carbon bonds .
- Preparation of Bioactive Compounds:
Pharmacological Applications
-
Antiviral and Antibacterial Agents:
- Research indicates that pyran derivatives, including those involving this compound, exhibit antiviral properties against diseases such as HIV and hepatitis C. The structural framework of pyrans has been linked to various biological activities, making them potential candidates for therapeutic agents .
- Anti-inflammatory Activities:
Agrochemical Applications
- Insecticides and Herbicides:
Case Study 1: Synthesis of Pyrrolotriazine-Based IRAK4 Inhibitors
In a study focused on developing IRAK4 inhibitors for potential cancer therapies, this compound was employed as a key building block. The reaction conditions were optimized to yield high purity compounds that demonstrated significant biological activity against cancer cell lines .
Case Study 2: Electrophilic Fluoroalkylthiolation
A novel method utilizing this compound in electrophilic fluoroalkylthiolation reactions was reported. This method allowed for the efficient synthesis of difluoromethylthiolated derivatives with potential applications in drug development. The scalability of the reaction was confirmed with yields reaching up to 75% on a gram scale .
Mechanism of Action
The mechanism of action of (3,6-Dihydro-2H-pyran-4-YL)boronic acid involves its ability to form stable complexes with various molecular targets. The boronic acid group can interact with diols and other nucleophiles, forming reversible covalent bonds. This property is exploited in the design of enzyme inhibitors and receptor modulators, where the compound can selectively bind to active sites or receptor domains, modulating their activity.
Comparison with Similar Compounds
Comparison with Similar Boronic Acid Derivatives
Structural and Physicochemical Properties
Table 1: Key Properties of Selected Boronic Acids
Key Observations :
- The dihydropyran ring in this compound enhances solubility compared to purely aromatic analogs like phenylboronic acid, though precipitation issues in polar media (e.g., RPMI culture medium) are common across many boronic acids .
- Its pKa is likely closer to physiological pH (~7.4) than 3-AcPBA, making it more suitable for biological applications .
Table 2: Antiproliferative and Enzymatic Inhibition Profiles
Key Observations :
- Bifunctional boronic acids like FL-166 demonstrate superior enzyme inhibition due to dual binding motifs, a feature absent in the target compound .
Table 3: Reactivity in Dynamic Combinatorial Chemistry (DCC) and Cross-Couplings
Key Observations :
Biological Activity
(3,6-Dihydro-2H-pyran-4-YL)boronic acid is a compound of significant interest in medicinal chemistry due to its unique structural features and biological activities. This article explores its biochemical properties, cellular effects, molecular mechanisms, and potential therapeutic applications.
This compound exhibits notable reactivity owing to its boronic acid functional group. This group allows the compound to interact with various biomolecules, particularly enzymes and proteins.
- Enzyme Inhibition : It has been shown to act as an inhibitor of serine proteases by forming covalent bonds with the active site serine residue. This interaction is critical for studying enzyme kinetics and developing novel enzyme inhibitors .
- Carbohydrate Binding : The compound can also interact with carbohydrate-binding proteins like lectins due to its affinity for diol-containing molecules .
2. Cellular Effects
The biological activity of this compound extends to various cellular processes:
- Cell Signaling Modulation : It influences cell signaling pathways, affecting gene expression and cellular metabolism. For example, it can inhibit proteasome activity, leading to the accumulation of ubiquitinated proteins and alterations in gene expression .
- Cell Cycle Impact : The compound has been implicated in affecting cell cycle progression and apoptosis, indicating its potential role in cancer therapy .
3. Molecular Mechanisms
The molecular mechanisms by which this compound exerts its effects include:
- Covalent Bond Formation : The compound's boron atom can form covalent bonds with active site residues of enzymes such as serine or threonine, leading to inhibition of enzymatic activity .
- Gene Expression Modulation : It can affect transcription factors and other regulatory proteins, thereby modulating gene expression .
4. Therapeutic Applications
Research indicates potential therapeutic applications for this compound across various fields:
- Cancer Therapy : Due to its ability to inhibit proteasomes and influence cell cycle dynamics, it is being studied as a potential anticancer agent .
- Antiviral Activity : Some studies suggest that derivatives of boronic acids exhibit antiviral properties by acting as competitive inhibitors of viral proteases .
Table 1: Summary of Biological Activities
Case Study: Proteasome Inhibition
In a study examining the effects of this compound on U266 cells (a human myeloma cell line), it was found that the compound effectively halted cell cycle progression at the G2/M phase. This led to significant growth inhibition in cancer cells, highlighting its potential application in oncology .
Q & A
Q. Basic: What are the optimal synthetic routes for (3,6-Dihydro-2H-pyran-4-YL)boronic acid?
Methodological Answer:
The compound is typically synthesized via its pinacol ester intermediate. A common route involves transesterification or hydrolysis of this compound pinacol ester (CAS 1142363-56-1) under mild acidic or basic conditions. For example, trifluoroacetic acid (TFA) in dichloromethane or aqueous NaOH can cleave the pinacol ester to yield the free boronic acid. The pinacol ester itself is synthesized via palladium-catalyzed Miyaura borylation of halogenated dihydropyran precursors .
Q. Basic: How do solubility and solvent choices impact experimental design with this boronic acid?
Methodological Answer:
Solubility in organic solvents is critical for reaction efficiency. Linear or cyclic ethers (e.g., dipropyl ether) are optimal for boronic acid reactions due to their polarity and ability to stabilize boronates. Chloroform is suitable for crystallization, while hydrocarbons (e.g., methylcyclohexane) aid in impurity removal. Aqueous buffers at physiological pH are preferred for glycoprotein interaction studies, but non-specific binding can occur; adjusting buffer composition (e.g., borate buffer at pH 8–9) enhances selectivity .
Q. Advanced: How does regioselective decarbonylation occur in palladium-catalyzed cross-coupling with this boronic acid?
Methodological Answer:
Regioselective decarbonylation is achieved through a concerted mechanism involving Pd-bipyridine coordination. The boronic acid acts as a directing group, enabling ortho-selective cross-coupling with nitriles. For example, 2-acetylbenzaldehyde does not decarbonylate without boronic acid coordination, highlighting the necessity of the boron-Pd interaction. Optimized conditions use Pd(OAc)₂, trifluoroacetate, and bipyridine ligands in anhydrous solvents .
Q. Advanced: What methodologies quantify glycoprotein interactions with this boronic acid?
Methodological Answer:
Surface plasmon resonance (SPR) with carboxymethyl dextran-coated gold substrates functionalized with boronic acid derivatives (e.g., AECPBA) is a robust approach. Binding affinity depends on terminal saccharide moieties (e.g., sialic acid vs. mannose). Non-specific interactions (e.g., hydrophobic effects) can be minimized using borate buffers at pH 8–9, which disrupt weak secondary interactions while retaining specific diol binding .
Q. Advanced: How do kinetic properties of diol binding influence real-time sensing applications?
Methodological Answer:
Stopped-flow fluorescence assays reveal binding kinetics between boronic acids and sugars (e.g., D-fructose, D-glucose). The kon values correlate with thermodynamic affinities (fructose > tagatose > mannose > glucose), confirming rapid equilibrium (<10 minutes). For real-time glucose monitoring, boronic acid chemosensors require fluorophores with microsecond response times to match the millisecond-scale binding kinetics .
Q. Advanced: What analytical techniques address challenges in characterizing boronic acid-functionalized peptides?
Methodological Answer:
MALDI-MS with in situ transesterification using 2,5-dihydroxybenzoic acid (DHB) as a matrix avoids boroxine formation. DHB esterifies boronic acids, enabling detection of polyboronic peptides (up to 5 boronic moieties) with 100% abundance. This method is compatible with alkyl- and arylboronic acids, facilitating sequencing of branched peptide libraries .
Q. Advanced: How does boronic acid oxidation impact drug stability in biological systems?
Methodological Answer:
Oxidation rates depend on ester substituents. Pinacol esters (t1/2 = 10 min) oxidize faster than neopentyl glycol esters (t1/2 = 27 min) under H2O2 exposure, independent of hydrolysis equilibrium. Relative diol affinity (pinacol > 2,3-butanediol > neopentyl glycol) inversely correlates with oxidation stability. Stability can be tuned by selecting diols with low ROS reactivity .
Q. Advanced: What structural features enhance thermal stability for flame-retardant applications?
Methodological Answer:
Aromatic boronic acids with electron-withdrawing groups (e.g., pyrene-1-boronic acid) exhibit exceptional thermal stability (>600°C). Thermogravimetric analysis (TGA) identifies degradation pathways: dehydration to boroxines occurs below 200°C, while oxidative decomposition dominates at higher temperatures. Functional groups like halides or nitro groups further stabilize the boron core .
Q. Advanced: How are boronic acids integrated into biosensors for bacterial detection?
Methodological Answer:
Boronic acid-functionalized carbon dots (B-CDs) bind glycolipids on Gram-positive bacterial surfaces via diol interactions. Fluorescence quenching or enhancement is quantified using confocal microscopy or flow cytometry. Selectivity is achieved by optimizing pH (7.4–8.5) and buffer ionic strength to minimize non-specific binding to Gram-negative bacteria .
Q. Basic: What computational tools aid in designing boronic acid-containing drug candidates?
Methodological Answer:
The Boronic Acid Navigator (https://bit.ly/boronics ) provides a curated database of 1,586 boronic acids with descriptors like clogP, H-bond donors, and topological polar surface area. Molecular docking and MD simulations prioritize compounds with high affinity for target proteins (e.g., proteases, glycoproteins). Bioisosteric replacement of carboxylates with boronic acids improves binding kinetics in rational drug design .
Properties
IUPAC Name |
3,6-dihydro-2H-pyran-4-ylboronic acid | |
---|---|---|
Source | PubChem | |
URL | https://pubchem.ncbi.nlm.nih.gov | |
Description | Data deposited in or computed by PubChem | |
InChI |
InChI=1S/C5H9BO3/c7-6(8)5-1-3-9-4-2-5/h1,7-8H,2-4H2 | |
Source | PubChem | |
URL | https://pubchem.ncbi.nlm.nih.gov | |
Description | Data deposited in or computed by PubChem | |
InChI Key |
YVWATSXVUOJOIG-UHFFFAOYSA-N | |
Source | PubChem | |
URL | https://pubchem.ncbi.nlm.nih.gov | |
Description | Data deposited in or computed by PubChem | |
Canonical SMILES |
B(C1=CCOCC1)(O)O | |
Source | PubChem | |
URL | https://pubchem.ncbi.nlm.nih.gov | |
Description | Data deposited in or computed by PubChem | |
Molecular Formula |
C5H9BO3 | |
Source | PubChem | |
URL | https://pubchem.ncbi.nlm.nih.gov | |
Description | Data deposited in or computed by PubChem | |
DSSTOX Substance ID |
DTXSID00635114 | |
Record name | 3,6-Dihydro-2H-pyran-4-ylboronic acid | |
Source | EPA DSSTox | |
URL | https://comptox.epa.gov/dashboard/DTXSID00635114 | |
Description | DSSTox provides a high quality public chemistry resource for supporting improved predictive toxicology. | |
Molecular Weight |
127.94 g/mol | |
Source | PubChem | |
URL | https://pubchem.ncbi.nlm.nih.gov | |
Description | Data deposited in or computed by PubChem | |
CAS No. |
1002127-60-7 | |
Record name | 3,6-Dihydro-2H-pyran-4-ylboronic acid | |
Source | EPA DSSTox | |
URL | https://comptox.epa.gov/dashboard/DTXSID00635114 | |
Description | DSSTox provides a high quality public chemistry resource for supporting improved predictive toxicology. | |
Retrosynthesis Analysis
AI-Powered Synthesis Planning: Our tool employs the Template_relevance Pistachio, Template_relevance Bkms_metabolic, Template_relevance Pistachio_ringbreaker, Template_relevance Reaxys, Template_relevance Reaxys_biocatalysis model, leveraging a vast database of chemical reactions to predict feasible synthetic routes.
One-Step Synthesis Focus: Specifically designed for one-step synthesis, it provides concise and direct routes for your target compounds, streamlining the synthesis process.
Accurate Predictions: Utilizing the extensive PISTACHIO, BKMS_METABOLIC, PISTACHIO_RINGBREAKER, REAXYS, REAXYS_BIOCATALYSIS database, our tool offers high-accuracy predictions, reflecting the latest in chemical research and data.
Strategy Settings
Precursor scoring | Relevance Heuristic |
---|---|
Min. plausibility | 0.01 |
Model | Template_relevance |
Template Set | Pistachio/Bkms_metabolic/Pistachio_ringbreaker/Reaxys/Reaxys_biocatalysis |
Top-N result to add to graph | 6 |
Feasible Synthetic Routes
Disclaimer and Information on In-Vitro Research Products
Please be aware that all articles and product information presented on BenchChem are intended solely for informational purposes. The products available for purchase on BenchChem are specifically designed for in-vitro studies, which are conducted outside of living organisms. In-vitro studies, derived from the Latin term "in glass," involve experiments performed in controlled laboratory settings using cells or tissues. It is important to note that these products are not categorized as medicines or drugs, and they have not received approval from the FDA for the prevention, treatment, or cure of any medical condition, ailment, or disease. We must emphasize that any form of bodily introduction of these products into humans or animals is strictly prohibited by law. It is essential to adhere to these guidelines to ensure compliance with legal and ethical standards in research and experimentation.