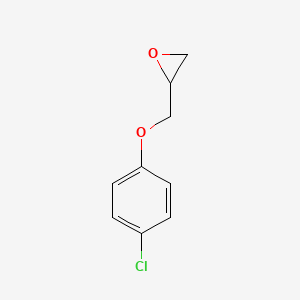
4-Chlorophenyl glycidyl ether
Overview
Description
4-Chlorophenyl glycidyl ether: is an organic compound with the molecular formula C9H9ClO2 and a molecular weight of 184.62 g/mol . It is also known by several other names, including 1,2-Epoxy-3-(4-chlorophenoxy)propane and 4-Chlorophenyl 2,3-epoxypropyl ether . This compound is characterized by the presence of an epoxide group and a chlorophenyl group, making it a versatile intermediate in organic synthesis .
Preparation Methods
Synthetic Routes and Reaction Conditions: 4-Chlorophenyl glycidyl ether can be synthesized through the Williamson Ether Synthesis . This method involves the reaction of 4-chlorophenol with epichlorohydrin in the presence of a base such as sodium hydroxide . The reaction proceeds via nucleophilic substitution, where the phenoxide ion attacks the epoxide ring of epichlorohydrin, resulting in the formation of this compound .
Industrial Production Methods: In industrial settings, the production of this compound typically involves the same Williamson Ether Synthesis but on a larger scale. The reaction is carried out in a controlled environment to ensure high yield and purity. The process may involve continuous flow reactors to optimize reaction conditions and minimize by-products .
Chemical Reactions Analysis
Types of Reactions: 4-Chlorophenyl glycidyl ether undergoes several types of chemical reactions, including:
Nucleophilic substitution: The epoxide ring can be opened by nucleophiles such as amines, alcohols, and thiols, leading to the formation of various substituted products.
Acidic cleavage: The ether bond can be cleaved by strong acids like hydrobromic acid (HBr) or hydroiodic acid (HI), resulting in the formation of 4-chlorophenol and glycidol.
Common Reagents and Conditions:
Nucleophilic substitution: Common reagents include amines, alcohols, and thiols.
Acidic cleavage: Strong acids such as HBr or HI are used, often in aqueous solutions, to cleave the ether bond.
Major Products:
Nucleophilic substitution: Depending on the nucleophile used, products can include various substituted ethers, amines, or thiols.
Acidic cleavage: The major products are 4-chlorophenol and glycidol.
Scientific Research Applications
4-Chlorophenyl glycidyl ether has a wide range of applications in scientific research, including:
Mechanism of Action
The mechanism of action of 4-chlorophenyl glycidyl ether primarily involves the epoxide ring-opening reactions . The epoxide group is highly reactive and can be attacked by nucleophiles, leading to the formation of various substituted products . This reactivity makes it a valuable intermediate in organic synthesis. The molecular targets and pathways involved depend on the specific nucleophile and reaction conditions used .
Comparison with Similar Compounds
- Glycidyl 4-methoxyphenyl ether
- Benzyl glycidyl ether
- 4-Chlorodiphenyl ether
Comparison: 4-Chlorophenyl glycidyl ether is unique due to the presence of both an epoxide group and a chlorophenyl group. This combination imparts distinct reactivity and makes it a versatile intermediate in organic synthesis. Compared to similar compounds like glycidyl 4-methoxyphenyl ether and benzyl glycidyl ether, this compound offers different reactivity patterns due to the electron-withdrawing effect of the chlorine atom .
Biological Activity
4-Chlorophenyl glycidyl ether (4-CPGE) is an epoxide compound that has garnered attention due to its biological activities, particularly its mutagenic properties and potential cytotoxic effects. This article synthesizes existing research findings, case studies, and relevant data tables to provide a comprehensive overview of the biological activity associated with this compound.
Chemical Structure and Properties
This compound is characterized by its epoxide group, which contributes to its reactivity and biological interactions. The chemical formula is CHClO, and it features a chlorinated phenyl ring attached to a glycidyl ether moiety. This structure allows for various metabolic transformations within biological systems.
Mutagenicity and Cytotoxicity
Research indicates that 4-CPGE exhibits significant mutagenic activity. A study evaluating various epoxides found that 4-CPGE was among the most mutagenic compounds tested, showing a high number of revertant colonies in bacterial assays (Table 1) . The mutagenic potential of 4-CPGE is believed to arise from its ability to form adducts with DNA, leading to mutations.
Table 1: Mutagenicity of Selected Epoxides
Compound | Revertants per Plate | % Reduction in Mutagenicity |
---|---|---|
This compound | 991 ± 86 | 0% |
Allylbenzene Oxide | 1883 ± 82 | 0% |
Styrene Oxide | 651 ± 44 | 65% |
As shown in Table 1, the mutagenic activity of 4-CPGE was significant, with no reduction in mutagenicity noted when combined with glutathione or cytosolic fractions .
The biological activity of 4-CPGE is largely attributed to its interaction with cellular components. Specifically, it has been shown to bind with glutathione in liver microsomes, which may influence its detoxification pathways . Additionally, the compound's epoxide group can react with nucleophiles in cells, leading to the formation of DNA adducts that can cause mutations.
Case Studies
Absorption and Metabolism
The absorption characteristics of glycidyl ethers indicate that they can penetrate biological membranes effectively. Studies have shown high percutaneous absorption rates for related compounds in animal models . The metabolic pathways typically involve hydrolysis by epoxide hydrolases, leading to less reactive diols which may be less harmful than their parent compounds.
Properties
IUPAC Name |
2-[(4-chlorophenoxy)methyl]oxirane | |
---|---|---|
Source | PubChem | |
URL | https://pubchem.ncbi.nlm.nih.gov | |
Description | Data deposited in or computed by PubChem | |
InChI |
InChI=1S/C9H9ClO2/c10-7-1-3-8(4-2-7)11-5-9-6-12-9/h1-4,9H,5-6H2 | |
Source | PubChem | |
URL | https://pubchem.ncbi.nlm.nih.gov | |
Description | Data deposited in or computed by PubChem | |
InChI Key |
KSLSZOOZWRMSAP-UHFFFAOYSA-N | |
Source | PubChem | |
URL | https://pubchem.ncbi.nlm.nih.gov | |
Description | Data deposited in or computed by PubChem | |
Canonical SMILES |
C1C(O1)COC2=CC=C(C=C2)Cl | |
Source | PubChem | |
URL | https://pubchem.ncbi.nlm.nih.gov | |
Description | Data deposited in or computed by PubChem | |
Molecular Formula |
C9H9ClO2 | |
Source | PubChem | |
URL | https://pubchem.ncbi.nlm.nih.gov | |
Description | Data deposited in or computed by PubChem | |
Molecular Weight |
184.62 g/mol | |
Source | PubChem | |
URL | https://pubchem.ncbi.nlm.nih.gov | |
Description | Data deposited in or computed by PubChem | |
CAS No. |
2212-05-7 | |
Record name | 2-[(4-Chlorophenoxy)methyl]oxirane | |
Source | CAS Common Chemistry | |
URL | https://commonchemistry.cas.org/detail?cas_rn=2212-05-7 | |
Description | CAS Common Chemistry is an open community resource for accessing chemical information. Nearly 500,000 chemical substances from CAS REGISTRY cover areas of community interest, including common and frequently regulated chemicals, and those relevant to high school and undergraduate chemistry classes. This chemical information, curated by our expert scientists, is provided in alignment with our mission as a division of the American Chemical Society. | |
Explanation | The data from CAS Common Chemistry is provided under a CC-BY-NC 4.0 license, unless otherwise stated. | |
Record name | Propane, 1-(p-chlorophenoxy)-2,3-epoxy- | |
Source | ChemIDplus | |
URL | https://pubchem.ncbi.nlm.nih.gov/substance/?source=chemidplus&sourceid=0002212057 | |
Description | ChemIDplus is a free, web search system that provides access to the structure and nomenclature authority files used for the identification of chemical substances cited in National Library of Medicine (NLM) databases, including the TOXNET system. | |
Record name | 2212-05-7 | |
Source | DTP/NCI | |
URL | https://dtp.cancer.gov/dtpstandard/servlet/dwindex?searchtype=NSC&outputformat=html&searchlist=60260 | |
Description | The NCI Development Therapeutics Program (DTP) provides services and resources to the academic and private-sector research communities worldwide to facilitate the discovery and development of new cancer therapeutic agents. | |
Explanation | Unless otherwise indicated, all text within NCI products is free of copyright and may be reused without our permission. Credit the National Cancer Institute as the source. | |
Record name | 4-chlorophenyl 2,3-epoxypropyl ether | |
Source | European Chemicals Agency (ECHA) | |
URL | https://echa.europa.eu/substance-information/-/substanceinfo/100.016.960 | |
Description | The European Chemicals Agency (ECHA) is an agency of the European Union which is the driving force among regulatory authorities in implementing the EU's groundbreaking chemicals legislation for the benefit of human health and the environment as well as for innovation and competitiveness. | |
Explanation | Use of the information, documents and data from the ECHA website is subject to the terms and conditions of this Legal Notice, and subject to other binding limitations provided for under applicable law, the information, documents and data made available on the ECHA website may be reproduced, distributed and/or used, totally or in part, for non-commercial purposes provided that ECHA is acknowledged as the source: "Source: European Chemicals Agency, http://echa.europa.eu/". Such acknowledgement must be included in each copy of the material. ECHA permits and encourages organisations and individuals to create links to the ECHA website under the following cumulative conditions: Links can only be made to webpages that provide a link to the Legal Notice page. | |
Synthesis routes and methods
Procedure details
Retrosynthesis Analysis
AI-Powered Synthesis Planning: Our tool employs the Template_relevance Pistachio, Template_relevance Bkms_metabolic, Template_relevance Pistachio_ringbreaker, Template_relevance Reaxys, Template_relevance Reaxys_biocatalysis model, leveraging a vast database of chemical reactions to predict feasible synthetic routes.
One-Step Synthesis Focus: Specifically designed for one-step synthesis, it provides concise and direct routes for your target compounds, streamlining the synthesis process.
Accurate Predictions: Utilizing the extensive PISTACHIO, BKMS_METABOLIC, PISTACHIO_RINGBREAKER, REAXYS, REAXYS_BIOCATALYSIS database, our tool offers high-accuracy predictions, reflecting the latest in chemical research and data.
Strategy Settings
Precursor scoring | Relevance Heuristic |
---|---|
Min. plausibility | 0.01 |
Model | Template_relevance |
Template Set | Pistachio/Bkms_metabolic/Pistachio_ringbreaker/Reaxys/Reaxys_biocatalysis |
Top-N result to add to graph | 6 |
Feasible Synthetic Routes
Disclaimer and Information on In-Vitro Research Products
Please be aware that all articles and product information presented on BenchChem are intended solely for informational purposes. The products available for purchase on BenchChem are specifically designed for in-vitro studies, which are conducted outside of living organisms. In-vitro studies, derived from the Latin term "in glass," involve experiments performed in controlled laboratory settings using cells or tissues. It is important to note that these products are not categorized as medicines or drugs, and they have not received approval from the FDA for the prevention, treatment, or cure of any medical condition, ailment, or disease. We must emphasize that any form of bodily introduction of these products into humans or animals is strictly prohibited by law. It is essential to adhere to these guidelines to ensure compliance with legal and ethical standards in research and experimentation.