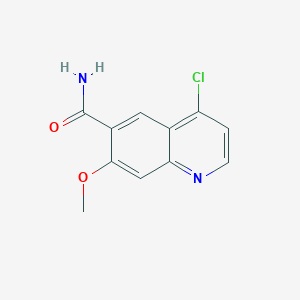
4-Chloro-7-methoxyquinoline-6-carboxamide
Overview
Description
4-Chloro-7-methoxyquinoline-6-carboxamide is a light yellow powder . It is used as an intermediate for synthesizing Lenvatinibis and is also used pharmaceutically for treating infection after burns .
Synthesis Analysis
The synthesis of 4-Chloro-7-methoxyquinoline-6-carboxamide involves a reaction with a molar ratio of hydrogen peroxide to Intermediate II of 1.05:1 . After the dropwise addition is completed, the reaction is returned to room temperature. Upon completion of the reaction, the reaction solution is added to ice water, filtered, washed with water, and dried to obtain 490 g of 4-chloro-7-methoxyquinoline-6-carboxamide with a yield of 91% .Molecular Structure Analysis
The molecular formula of 4-Chloro-7-methoxyquinoline-6-carboxamide is C11H9ClN2O2 . It has a molecular weight of 236.65 .Chemical Reactions Analysis
4-Chloro-7-methoxyquinoline-6-carboxamide is used as a reagent in the preparation of naphthamides as inhibitors of vascular endothelial growth factor (VEGF) receptor tyrosine kinase .Physical And Chemical Properties Analysis
4-Chloro-7-methoxyquinoline-6-carboxamide is a solid, off-white to light beige in color . It has a melting point of >205°C (dec.), a boiling point of 426.7±45.0 °C (Predicted), and a density of 1.380±0.06 g/cm3 (Predicted) . It is slightly soluble in DMSO and Methanol .Scientific Research Applications
Pharmaceutical Intermediate
This compound is used as a pharmaceutical intermediate in the preparation of Lenvatinib , which is an oral multi-receptor tyrosine kinase inhibitor. Lenvatinib is approved by the FDA for the treatment of various cancers such as differentiated thyroid cancer (DTC), unresectable hepatocellular carcinoma (HCC), and advanced renal cell carcinoma (RCC) .
Organic Synthesis
It is also used as an organic synthesis intermediate, which can be utilized in laboratory research and development processes, as well as in chemical production processes .
Synthesis Methodology Research
Research into new methods for synthesizing key intermediates for anti-cancer drugs like Lenvatinib includes using 4-Chloro-7-methoxyquinoline-6-carboxamide . This involves exploring catalysts and reaction conditions to improve yield and efficiency .
Chemical Research
The compound is involved in chemical research related to nitration reactions and the development of efficient strategies for total synthesis of complex molecules .
Analytical Chemistry
In analytical chemistry, it may be used to develop standards and reagents for quality control and validation of analytical methods related to pharmaceuticals .
Safety And Hazards
The compound is labeled with the GHS07 pictogram and has the signal word "Warning" . The hazard statements include H302, H315, H319, H335 . Precautionary statements include P261, P305, P338, P351 . It is recommended to avoid breathing dust and contact with skin and eyes. Protective clothing, gloves, safety glasses, and a dust respirator should be worn .
properties
IUPAC Name |
4-chloro-7-methoxyquinoline-6-carboxamide | |
---|---|---|
Source | PubChem | |
URL | https://pubchem.ncbi.nlm.nih.gov | |
Description | Data deposited in or computed by PubChem | |
InChI |
InChI=1S/C11H9ClN2O2/c1-16-10-5-9-6(4-7(10)11(13)15)8(12)2-3-14-9/h2-5H,1H3,(H2,13,15) | |
Source | PubChem | |
URL | https://pubchem.ncbi.nlm.nih.gov | |
Description | Data deposited in or computed by PubChem | |
InChI Key |
ZBTVNIDMGKZSGC-UHFFFAOYSA-N | |
Source | PubChem | |
URL | https://pubchem.ncbi.nlm.nih.gov | |
Description | Data deposited in or computed by PubChem | |
Canonical SMILES |
COC1=CC2=NC=CC(=C2C=C1C(=O)N)Cl | |
Source | PubChem | |
URL | https://pubchem.ncbi.nlm.nih.gov | |
Description | Data deposited in or computed by PubChem | |
Molecular Formula |
C11H9ClN2O2 | |
Source | PubChem | |
URL | https://pubchem.ncbi.nlm.nih.gov | |
Description | Data deposited in or computed by PubChem | |
DSSTOX Substance ID |
DTXSID10629105 | |
Record name | 4-Chloro-7-methoxyquinoline-6-carboxamide | |
Source | EPA DSSTox | |
URL | https://comptox.epa.gov/dashboard/DTXSID10629105 | |
Description | DSSTox provides a high quality public chemistry resource for supporting improved predictive toxicology. | |
Molecular Weight |
236.65 g/mol | |
Source | PubChem | |
URL | https://pubchem.ncbi.nlm.nih.gov | |
Description | Data deposited in or computed by PubChem | |
Product Name |
4-Chloro-7-methoxyquinoline-6-carboxamide | |
CAS RN |
417721-36-9 | |
Record name | 4-Chloro-7-methoxyquinoline-6-carboxamide | |
Source | EPA DSSTox | |
URL | https://comptox.epa.gov/dashboard/DTXSID10629105 | |
Description | DSSTox provides a high quality public chemistry resource for supporting improved predictive toxicology. | |
Synthesis routes and methods
Procedure details
Retrosynthesis Analysis
AI-Powered Synthesis Planning: Our tool employs the Template_relevance Pistachio, Template_relevance Bkms_metabolic, Template_relevance Pistachio_ringbreaker, Template_relevance Reaxys, Template_relevance Reaxys_biocatalysis model, leveraging a vast database of chemical reactions to predict feasible synthetic routes.
One-Step Synthesis Focus: Specifically designed for one-step synthesis, it provides concise and direct routes for your target compounds, streamlining the synthesis process.
Accurate Predictions: Utilizing the extensive PISTACHIO, BKMS_METABOLIC, PISTACHIO_RINGBREAKER, REAXYS, REAXYS_BIOCATALYSIS database, our tool offers high-accuracy predictions, reflecting the latest in chemical research and data.
Strategy Settings
Precursor scoring | Relevance Heuristic |
---|---|
Min. plausibility | 0.01 |
Model | Template_relevance |
Template Set | Pistachio/Bkms_metabolic/Pistachio_ringbreaker/Reaxys/Reaxys_biocatalysis |
Top-N result to add to graph | 6 |
Feasible Synthetic Routes
Q & A
Q1: The provided research paper describes an optimized synthesis route for Lenvatinib involving 4-Chloro-7-methoxyquinoline-6-carboxamide. What are the key advantages of this specific synthetic route compared to other potential methods?
A: The optimized synthetic route presented in the research paper [] offers several advantages:
Disclaimer and Information on In-Vitro Research Products
Please be aware that all articles and product information presented on BenchChem are intended solely for informational purposes. The products available for purchase on BenchChem are specifically designed for in-vitro studies, which are conducted outside of living organisms. In-vitro studies, derived from the Latin term "in glass," involve experiments performed in controlled laboratory settings using cells or tissues. It is important to note that these products are not categorized as medicines or drugs, and they have not received approval from the FDA for the prevention, treatment, or cure of any medical condition, ailment, or disease. We must emphasize that any form of bodily introduction of these products into humans or animals is strictly prohibited by law. It is essential to adhere to these guidelines to ensure compliance with legal and ethical standards in research and experimentation.