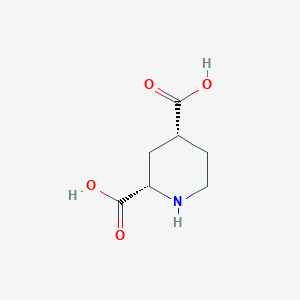
cis-Piperidine-2,4-dicarboxylic acid
- Click on QUICK INQUIRY to receive a quote from our team of experts.
- With the quality product at a COMPETITIVE price, you can focus more on your research.
Description
Cis-Piperidine-2,4-dicarboxylic acid is a useful research compound. Its molecular formula is C7H11NO4 and its molecular weight is 173.17 g/mol. The purity is usually 95%.
BenchChem offers high-quality this compound suitable for many research applications. Different packaging options are available to accommodate customers' requirements. Please inquire for more information about this compound including the price, delivery time, and more detailed information at info@benchchem.com.
Mechanism of Action
Target of Action
The primary targets of cis-Piperidine-2,4-dicarboxylic acid are ionotropic receptors, specifically NMDA (N-methyl-D-aspartate), AMPA (α-amino-3-hydroxy-5-methyl-4-isoxazolepropionic acid), and kainate receptors . These receptors play a crucial role in the central nervous system, mediating synaptic transmission and plasticity, which are essential for learning and memory .
Mode of Action
This compound acts as a general ionotropic receptor antagonist, blocking the responses mediated by NMDA, AMPA, and kainate receptors . Interestingly, it also acts as a partial NMDA receptor agonist in certain models, such as the in vitro rat cerebellar cGMP model .
Biochemical Pathways
By blocking the NMDA, AMPA, and kainate receptors, this compound inhibits the excitatory synaptic transmissions in the central nervous system . This can affect various downstream biochemical pathways involved in neural communication and plasticity.
Pharmacokinetics
It is soluble in water, which may influence its bioavailability .
Result of Action
The molecular and cellular effects of this compound’s action primarily involve the modulation of neural activity. By blocking excitatory synaptic transmissions, it can influence neural signaling and potentially impact processes such as learning and memory .
Biochemical Analysis
Biochemical Properties
cis-Piperidine-2,4-dicarboxylic acid plays a significant role in biochemical reactions by interacting with various ionotropic receptors. It is known to block NMDA, AMPA, and kainate receptor-mediated responses, which are crucial for excitatory synaptic transmission in the central nervous system. The compound’s interaction with these receptors involves binding to the receptor sites, thereby inhibiting their activity. This inhibition can modulate synaptic plasticity and neurotransmission, which are essential for learning and memory processes .
Cellular Effects
The effects of this compound on various cell types and cellular processes are profound. In neuronal cells, it influences cell function by modulating cell signaling pathways, particularly those involving NMDA, AMPA, and kainate receptors. This modulation can lead to changes in gene expression and cellular metabolism. For instance, the inhibition of NMDA receptors by this compound can reduce calcium influx, thereby affecting downstream signaling pathways and gene transcription .
Molecular Mechanism
The molecular mechanism of action of this compound involves its binding interactions with ionotropic receptors. By binding to the receptor sites, it inhibits the activity of NMDA, AMPA, and kainate receptors. This inhibition can prevent the excitatory neurotransmitter glutamate from activating these receptors, thereby reducing excitatory synaptic transmission. Additionally, as a partial agonist for NMDA receptors, this compound can activate these receptors to a lesser extent, leading to a partial response .
Temporal Effects in Laboratory Settings
In laboratory settings, the effects of this compound can change over time. The compound’s stability and degradation are critical factors that influence its long-term effects on cellular function. Studies have shown that this compound can maintain its activity for extended periods when stored under appropriate conditions. Prolonged exposure to light or extreme temperatures can lead to its degradation, reducing its efficacy in biochemical assays .
Dosage Effects in Animal Models
The effects of this compound vary with different dosages in animal models. At lower doses, the compound can effectively inhibit ionotropic receptor activity without causing significant adverse effects. At higher doses, this compound may exhibit toxic effects, including neurotoxicity and disruptions in normal cellular function. These threshold effects highlight the importance of dosage optimization in experimental studies .
Metabolic Pathways
This compound is involved in various metabolic pathways, particularly those related to neurotransmitter metabolism. It interacts with enzymes and cofactors that regulate the synthesis and degradation of neurotransmitters. For example, the compound’s inhibition of NMDA receptors can affect the metabolism of glutamate, a key excitatory neurotransmitter. This interaction can lead to changes in metabolic flux and metabolite levels, impacting overall cellular metabolism .
Transport and Distribution
The transport and distribution of this compound within cells and tissues are mediated by specific transporters and binding proteins. These interactions facilitate the compound’s localization and accumulation in target tissues, such as the brain. The compound’s ability to cross the blood-brain barrier is particularly important for its efficacy in modulating central nervous system functions .
Subcellular Localization
The subcellular localization of this compound is crucial for its activity and function. The compound is directed to specific compartments or organelles within the cell, where it can exert its effects. Targeting signals and post-translational modifications play a role in directing this compound to these locations. For instance, its localization to synaptic sites allows it to effectively modulate synaptic transmission and plasticity .
Properties
IUPAC Name |
(2S,4R)-piperidine-2,4-dicarboxylic acid |
Source
|
---|---|---|
Source | PubChem | |
URL | https://pubchem.ncbi.nlm.nih.gov | |
Description | Data deposited in or computed by PubChem | |
InChI |
InChI=1S/C7H11NO4/c9-6(10)4-1-2-8-5(3-4)7(11)12/h4-5,8H,1-3H2,(H,9,10)(H,11,12)/t4-,5+/m1/s1 |
Source
|
Source | PubChem | |
URL | https://pubchem.ncbi.nlm.nih.gov | |
Description | Data deposited in or computed by PubChem | |
InChI Key |
WXUOEIRUBILDKO-UHNVWZDZSA-N |
Source
|
Source | PubChem | |
URL | https://pubchem.ncbi.nlm.nih.gov | |
Description | Data deposited in or computed by PubChem | |
Canonical SMILES |
C1CNC(CC1C(=O)O)C(=O)O |
Source
|
Source | PubChem | |
URL | https://pubchem.ncbi.nlm.nih.gov | |
Description | Data deposited in or computed by PubChem | |
Isomeric SMILES |
C1CN[C@@H](C[C@@H]1C(=O)O)C(=O)O |
Source
|
Source | PubChem | |
URL | https://pubchem.ncbi.nlm.nih.gov | |
Description | Data deposited in or computed by PubChem | |
Molecular Formula |
C7H11NO4 |
Source
|
Source | PubChem | |
URL | https://pubchem.ncbi.nlm.nih.gov | |
Description | Data deposited in or computed by PubChem | |
Molecular Weight |
173.17 g/mol |
Source
|
Source | PubChem | |
URL | https://pubchem.ncbi.nlm.nih.gov | |
Description | Data deposited in or computed by PubChem | |
Disclaimer and Information on In-Vitro Research Products
Please be aware that all articles and product information presented on BenchChem are intended solely for informational purposes. The products available for purchase on BenchChem are specifically designed for in-vitro studies, which are conducted outside of living organisms. In-vitro studies, derived from the Latin term "in glass," involve experiments performed in controlled laboratory settings using cells or tissues. It is important to note that these products are not categorized as medicines or drugs, and they have not received approval from the FDA for the prevention, treatment, or cure of any medical condition, ailment, or disease. We must emphasize that any form of bodily introduction of these products into humans or animals is strictly prohibited by law. It is essential to adhere to these guidelines to ensure compliance with legal and ethical standards in research and experimentation.