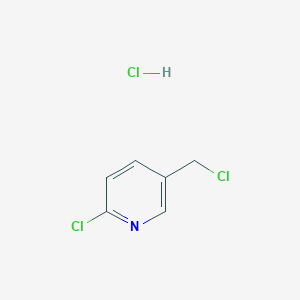
2-Chloro-5-chloromethylpyridine hydrochloride
Overview
Description
2-Chloro-5-chloromethylpyridine hydrochloride is an important chemical compound widely used as an intermediate in the synthesis of various pharmaceuticals and agrochemicals. It is particularly significant in the production of insecticides such as imidacloprid, which targets insect nicotinic acetylcholine receptors .
Preparation Methods
Synthetic Routes and Reaction Conditions
The synthesis of 2-Chloro-5-chloromethylpyridine hydrochloride typically involves the chlorination of 2-chloro-5-methylpyridine. One common method includes the reaction of 2-chloro-5-methylpyridine with chlorine gas in the presence of a catalyst such as iron(III) chloride at elevated temperatures . Another method involves the use of phosgene in the production process, which significantly reduces waste and environmental impact .
Industrial Production Methods
Industrial production of this compound often employs large-scale chlorination processes. These processes are optimized to ensure high yield and purity of the final product. The use of advanced catalytic systems and controlled reaction conditions are crucial to achieving efficient production .
Chemical Reactions Analysis
Types of Reactions
2-Chloro-5-chloromethylpyridine hydrochloride undergoes various chemical reactions, including:
Substitution Reactions: It can participate in nucleophilic substitution reactions where the chlorine atom is replaced by other nucleophiles.
Oxidation Reactions: The compound can be oxidized to form corresponding pyridine N-oxides.
Reduction Reactions: It can be reduced to form 2-chloro-5-methylpyridine.
Common Reagents and Conditions
Substitution: Common reagents include sodium hydroxide, potassium hydroxide, and various amines.
Oxidation: Reagents such as hydrogen peroxide or peracids are used.
Reduction: Catalytic hydrogenation using palladium or platinum catalysts is common.
Major Products Formed
Substitution: Products include various substituted pyridines.
Oxidation: Pyridine N-oxides.
Reduction: 2-Chloro-5-methylpyridine.
Scientific Research Applications
2-Chloro-5-chloromethylpyridine hydrochloride has numerous applications in scientific research:
Chemistry: It is used as a building block in the synthesis of complex organic molecules.
Biology: The compound is used in the study of enzyme inhibitors and receptor binding studies.
Medicine: It serves as an intermediate in the synthesis of pharmaceuticals, particularly in the development of insecticides and antimicrobial agents.
Mechanism of Action
The mechanism of action of 2-Chloro-5-chloromethylpyridine hydrochloride primarily involves its role as an intermediate in the synthesis of insecticides. For example, in the case of imidacloprid, the compound targets insect nicotinic acetylcholine receptors, leading to paralysis and death of the insect. This selective action on insect receptors makes it highly effective while minimizing impact on non-target species .
Comparison with Similar Compounds
Similar Compounds
- 2-Chloro-5-methylpyridine
- 2-Chloro-5-bromomethylpyridine
- 2-Chloro-5-fluoromethylpyridine
Uniqueness
2-Chloro-5-chloromethylpyridine hydrochloride is unique due to its specific structure that allows for selective targeting of insect nicotinic acetylcholine receptors. This specificity enhances its effectiveness as an insecticide intermediate, making it a preferred choice in the synthesis of compounds like imidacloprid .
Properties
IUPAC Name |
2-chloro-5-(chloromethyl)pyridine;hydrochloride | |
---|---|---|
Source | PubChem | |
URL | https://pubchem.ncbi.nlm.nih.gov | |
Description | Data deposited in or computed by PubChem | |
InChI |
InChI=1S/C6H5Cl2N.ClH/c7-3-5-1-2-6(8)9-4-5;/h1-2,4H,3H2;1H | |
Source | PubChem | |
URL | https://pubchem.ncbi.nlm.nih.gov | |
Description | Data deposited in or computed by PubChem | |
InChI Key |
ZHEXGKWVNRSSLH-UHFFFAOYSA-N | |
Source | PubChem | |
URL | https://pubchem.ncbi.nlm.nih.gov | |
Description | Data deposited in or computed by PubChem | |
Canonical SMILES |
C1=CC(=NC=C1CCl)Cl.Cl | |
Source | PubChem | |
URL | https://pubchem.ncbi.nlm.nih.gov | |
Description | Data deposited in or computed by PubChem | |
Molecular Formula |
C6H6Cl3N | |
Source | PubChem | |
URL | https://pubchem.ncbi.nlm.nih.gov | |
Description | Data deposited in or computed by PubChem | |
Molecular Weight |
198.5 g/mol | |
Source | PubChem | |
URL | https://pubchem.ncbi.nlm.nih.gov | |
Description | Data deposited in or computed by PubChem | |
Synthesis routes and methods
Procedure details
Retrosynthesis Analysis
AI-Powered Synthesis Planning: Our tool employs the Template_relevance Pistachio, Template_relevance Bkms_metabolic, Template_relevance Pistachio_ringbreaker, Template_relevance Reaxys, Template_relevance Reaxys_biocatalysis model, leveraging a vast database of chemical reactions to predict feasible synthetic routes.
One-Step Synthesis Focus: Specifically designed for one-step synthesis, it provides concise and direct routes for your target compounds, streamlining the synthesis process.
Accurate Predictions: Utilizing the extensive PISTACHIO, BKMS_METABOLIC, PISTACHIO_RINGBREAKER, REAXYS, REAXYS_BIOCATALYSIS database, our tool offers high-accuracy predictions, reflecting the latest in chemical research and data.
Strategy Settings
Precursor scoring | Relevance Heuristic |
---|---|
Min. plausibility | 0.01 |
Model | Template_relevance |
Template Set | Pistachio/Bkms_metabolic/Pistachio_ringbreaker/Reaxys/Reaxys_biocatalysis |
Top-N result to add to graph | 6 |
Feasible Synthetic Routes
Disclaimer and Information on In-Vitro Research Products
Please be aware that all articles and product information presented on BenchChem are intended solely for informational purposes. The products available for purchase on BenchChem are specifically designed for in-vitro studies, which are conducted outside of living organisms. In-vitro studies, derived from the Latin term "in glass," involve experiments performed in controlled laboratory settings using cells or tissues. It is important to note that these products are not categorized as medicines or drugs, and they have not received approval from the FDA for the prevention, treatment, or cure of any medical condition, ailment, or disease. We must emphasize that any form of bodily introduction of these products into humans or animals is strictly prohibited by law. It is essential to adhere to these guidelines to ensure compliance with legal and ethical standards in research and experimentation.