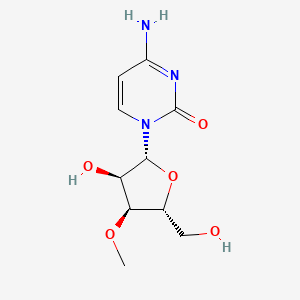
3'-O-Methylcytidine
Overview
Description
3’-O-Methylcytidine is a modified nucleoside, specifically a pyrimidine nucleoside, with the chemical formula C10H15N3O5. It is characterized by the presence of a methyl group attached to the 3’ oxygen of the ribose sugar. This compound is found in various RNA species and plays a significant role in the regulation of RNA function and stability .
Mechanism of Action
Target of Action
3’-O-Methylcytidine is a cytidine analog . The primary targets of 3’-O-Methylcytidine are DNA methyltransferases . These enzymes play a crucial role in the methylation of DNA, a process that is vital for gene expression and regulation .
Mode of Action
3’-O-Methylcytidine interacts with its targets, the DNA methyltransferases, by inhibiting their function . This inhibition occurs because 3’-O-Methylcytidine, being a cytidine analog, can mimic the natural substrate of these enzymes, thereby blocking their normal activity .
Biochemical Pathways
The inhibition of DNA methyltransferases by 3’-O-Methylcytidine affects the methylation pathways of DNA . This can lead to changes in gene expression, as DNA methylation is a key mechanism for controlling gene activity . Additionally, 3’-O-Methylcytidine has been found in cellular RNAs, influencing their biogenesis, stability, and function .
Result of Action
The inhibition of DNA methyltransferases by 3’-O-Methylcytidine can lead to changes in gene expression . This can have various effects at the molecular and cellular levels, potentially leading to anti-metabolic and anti-tumor activities . In cellular RNAs, 3’-O-Methylcytidine can influence their biogenesis, stability, and function .
Biochemical Analysis
Biochemical Properties
3’-O-Methylcytidine is involved in several biochemical reactions, particularly those related to RNA metabolism. It interacts with various enzymes, proteins, and other biomolecules. One of the key interactions is with DNA methyltransferases, which are enzymes responsible for adding methyl groups to DNA. 3’-O-Methylcytidine has been shown to inhibit these enzymes, thereby affecting DNA methylation patterns . This inhibition can lead to changes in gene expression and has potential anti-metabolic and anti-tumor activities . Additionally, 3’-O-Methylcytidine can interact with RNA molecules, influencing their stability and function.
Cellular Effects
The effects of 3’-O-Methylcytidine on cells are diverse and significant. It can influence cell function by affecting cell signaling pathways, gene expression, and cellular metabolism. For instance, 3’-O-Methylcytidine has been shown to inhibit DNA methyltransferases, leading to changes in DNA methylation patterns and subsequent alterations in gene expression . This can impact various cellular processes, including cell cycle regulation, apoptosis, and differentiation. Furthermore, 3’-O-Methylcytidine can affect the stability and function of RNA molecules, thereby influencing protein synthesis and other RNA-dependent processes.
Molecular Mechanism
The molecular mechanism of 3’-O-Methylcytidine involves its interaction with DNA methyltransferases and RNA molecules. By inhibiting DNA methyltransferases, 3’-O-Methylcytidine prevents the addition of methyl groups to DNA, leading to changes in DNA methylation patterns . This can result in altered gene expression and subsequent changes in cellular function. Additionally, 3’-O-Methylcytidine can bind to RNA molecules, affecting their stability and function. This interaction can influence RNA processing, translation, and degradation, thereby impacting protein synthesis and other RNA-dependent processes.
Temporal Effects in Laboratory Settings
In laboratory settings, the effects of 3’-O-Methylcytidine can change over time. The stability and degradation of 3’-O-Methylcytidine are important factors to consider. It has been shown that 3’-O-Methylcytidine is relatively stable under certain conditions, but it can degrade over time, especially when exposed to light and heat . Long-term effects of 3’-O-Methylcytidine on cellular function have been observed in both in vitro and in vivo studies. These effects include changes in gene expression, cell cycle regulation, and apoptosis, which can persist even after the compound has degraded.
Dosage Effects in Animal Models
The effects of 3’-O-Methylcytidine can vary with different dosages in animal models. At low doses, 3’-O-Methylcytidine has been shown to inhibit DNA methyltransferases and alter gene expression without causing significant toxicity . At higher doses, 3’-O-Methylcytidine can have toxic effects, including cytotoxicity and apoptosis. These adverse effects are likely due to the inhibition of DNA methyltransferases and subsequent changes in gene expression. Threshold effects have also been observed, where a certain dosage is required to achieve a significant biological effect.
Metabolic Pathways
3’-O-Methylcytidine is involved in several metabolic pathways, particularly those related to RNA metabolism. It interacts with enzymes such as DNA methyltransferases, which are responsible for adding methyl groups to DNA . By inhibiting these enzymes, 3’-O-Methylcytidine can affect DNA methylation patterns and subsequent gene expression. Additionally, 3’-O-Methylcytidine can influence metabolic flux and metabolite levels by affecting RNA stability and function. This can impact various cellular processes, including protein synthesis and RNA-dependent metabolic pathways.
Transport and Distribution
The transport and distribution of 3’-O-Methylcytidine within cells and tissues are important factors to consider. 3’-O-Methylcytidine can be transported into cells via nucleoside transporters, which are proteins responsible for the uptake of nucleosides . Once inside the cell, 3’-O-Methylcytidine can interact with various biomolecules, including DNA methyltransferases and RNA molecules. The distribution of 3’-O-Methylcytidine within the cell can affect its localization and accumulation, thereby influencing its biological activity.
Subcellular Localization
The subcellular localization of 3’-O-Methylcytidine can affect its activity and function. 3’-O-Methylcytidine can be localized to specific compartments or organelles within the cell, depending on its interactions with targeting signals and post-translational modifications . For example, 3’-O-Methylcytidine can be directed to the nucleus, where it can interact with DNA methyltransferases and affect DNA methylation patterns. Additionally, 3’-O-Methylcytidine can be localized to the cytoplasm, where it can interact with RNA molecules and influence RNA stability and function.
Preparation Methods
Synthetic Routes and Reaction Conditions: The synthesis of 3’-O-Methylcytidine typically involves the methylation of cytidine. One common method is the reaction of cytidine with methyl iodide in the presence of a base such as sodium hydride. The reaction is carried out in an aprotic solvent like dimethylformamide (DMF) at elevated temperatures .
Industrial Production Methods: Industrial production of 3’-O-Methylcytidine may involve enzymatic methods where specific methyltransferases catalyze the methylation of cytidine. This approach is advantageous due to its specificity and efficiency, reducing the need for extensive purification steps .
Chemical Reactions Analysis
Types of Reactions: 3’-O-Methylcytidine can undergo various chemical reactions, including:
Oxidation: This reaction can lead to the formation of oxidized derivatives, which may have different biological activities.
Reduction: Reduction reactions can modify the nucleoside, potentially altering its function.
Substitution: Substitution reactions, particularly nucleophilic substitutions, can introduce different functional groups into the molecule.
Common Reagents and Conditions:
Oxidation: Common oxidizing agents include hydrogen peroxide and potassium permanganate.
Reduction: Reducing agents such as sodium borohydride or lithium aluminum hydride are often used.
Substitution: Nucleophilic substitution reactions may involve reagents like sodium azide or thiols.
Major Products Formed: The major products formed from these reactions depend on the specific conditions and reagents used. For example, oxidation may yield hydroxylated derivatives, while substitution reactions can produce a variety of modified nucleosides .
Scientific Research Applications
3’-O-Methylcytidine has several important applications in scientific research:
Chemistry: It is used as a building block in the synthesis of modified RNA molecules, which are essential for studying RNA structure and function.
Biology: The compound is involved in the regulation of gene expression and RNA stability, making it a valuable tool for understanding cellular processes.
Medicine: Modified nucleosides like 3’-O-Methylcytidine are explored for their potential therapeutic applications, including antiviral and anticancer treatments.
Industry: In the biotechnology industry, 3’-O-Methylcytidine is used in the production of synthetic RNA for research and therapeutic purposes
Comparison with Similar Compounds
2’-O-Methylcytidine: Another methylated nucleoside with the methyl group at the 2’ position of the ribose sugar.
5-Methylcytidine: A methylated nucleoside with the methyl group attached to the 5 position of the cytosine base.
N4-Methylcytidine: A nucleoside with a methyl group at the N4 position of the cytosine base.
Uniqueness: 3’-O-Methylcytidine is unique due to its specific methylation at the 3’ position of the ribose sugar. This modification can have distinct effects on RNA structure and function compared to other methylated nucleosides. Its unique properties make it a valuable tool for studying RNA biology and developing therapeutic applications .
Properties
IUPAC Name |
4-amino-1-[(2R,3R,4S,5R)-3-hydroxy-5-(hydroxymethyl)-4-methoxyoxolan-2-yl]pyrimidin-2-one | |
---|---|---|
Source | PubChem | |
URL | https://pubchem.ncbi.nlm.nih.gov | |
Description | Data deposited in or computed by PubChem | |
InChI |
InChI=1S/C10H15N3O5/c1-17-8-5(4-14)18-9(7(8)15)13-3-2-6(11)12-10(13)16/h2-3,5,7-9,14-15H,4H2,1H3,(H2,11,12,16)/t5-,7-,8-,9-/m1/s1 | |
Source | PubChem | |
URL | https://pubchem.ncbi.nlm.nih.gov | |
Description | Data deposited in or computed by PubChem | |
InChI Key |
RZJCFLSPBDUNDH-ZOQUXTDFSA-N | |
Source | PubChem | |
URL | https://pubchem.ncbi.nlm.nih.gov | |
Description | Data deposited in or computed by PubChem | |
Canonical SMILES |
COC1C(OC(C1O)N2C=CC(=NC2=O)N)CO | |
Source | PubChem | |
URL | https://pubchem.ncbi.nlm.nih.gov | |
Description | Data deposited in or computed by PubChem | |
Isomeric SMILES |
CO[C@@H]1[C@H](O[C@H]([C@@H]1O)N2C=CC(=NC2=O)N)CO | |
Source | PubChem | |
URL | https://pubchem.ncbi.nlm.nih.gov | |
Description | Data deposited in or computed by PubChem | |
Molecular Formula |
C10H15N3O5 | |
Source | PubChem | |
URL | https://pubchem.ncbi.nlm.nih.gov | |
Description | Data deposited in or computed by PubChem | |
DSSTOX Substance ID |
DTXSID90595374 | |
Record name | 3'-O-Methylcytidine | |
Source | EPA DSSTox | |
URL | https://comptox.epa.gov/dashboard/DTXSID90595374 | |
Description | DSSTox provides a high quality public chemistry resource for supporting improved predictive toxicology. | |
Molecular Weight |
257.24 g/mol | |
Source | PubChem | |
URL | https://pubchem.ncbi.nlm.nih.gov | |
Description | Data deposited in or computed by PubChem | |
CAS No. |
20594-00-7 | |
Record name | 3'-O-Methylcytidine | |
Source | EPA DSSTox | |
URL | https://comptox.epa.gov/dashboard/DTXSID90595374 | |
Description | DSSTox provides a high quality public chemistry resource for supporting improved predictive toxicology. | |
Retrosynthesis Analysis
AI-Powered Synthesis Planning: Our tool employs the Template_relevance Pistachio, Template_relevance Bkms_metabolic, Template_relevance Pistachio_ringbreaker, Template_relevance Reaxys, Template_relevance Reaxys_biocatalysis model, leveraging a vast database of chemical reactions to predict feasible synthetic routes.
One-Step Synthesis Focus: Specifically designed for one-step synthesis, it provides concise and direct routes for your target compounds, streamlining the synthesis process.
Accurate Predictions: Utilizing the extensive PISTACHIO, BKMS_METABOLIC, PISTACHIO_RINGBREAKER, REAXYS, REAXYS_BIOCATALYSIS database, our tool offers high-accuracy predictions, reflecting the latest in chemical research and data.
Strategy Settings
Precursor scoring | Relevance Heuristic |
---|---|
Min. plausibility | 0.01 |
Model | Template_relevance |
Template Set | Pistachio/Bkms_metabolic/Pistachio_ringbreaker/Reaxys/Reaxys_biocatalysis |
Top-N result to add to graph | 6 |
Feasible Synthetic Routes
Disclaimer and Information on In-Vitro Research Products
Please be aware that all articles and product information presented on BenchChem are intended solely for informational purposes. The products available for purchase on BenchChem are specifically designed for in-vitro studies, which are conducted outside of living organisms. In-vitro studies, derived from the Latin term "in glass," involve experiments performed in controlled laboratory settings using cells or tissues. It is important to note that these products are not categorized as medicines or drugs, and they have not received approval from the FDA for the prevention, treatment, or cure of any medical condition, ailment, or disease. We must emphasize that any form of bodily introduction of these products into humans or animals is strictly prohibited by law. It is essential to adhere to these guidelines to ensure compliance with legal and ethical standards in research and experimentation.