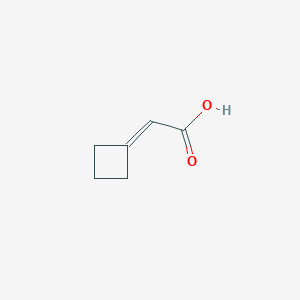
2-Cyclobutylideneacetic acid
Overview
Description
2-Cyclobutylideneacetic acid is an organic compound with the molecular formula C6H8O2 and a molecular weight of 112.13 g/mol It is characterized by a cyclobutylidene group attached to an acetic acid moiety
Preparation Methods
Synthetic Routes and Reaction Conditions: The synthesis of 2-Cyclobutylideneacetic acid typically involves the Horner–Wadsworth–Emmons reaction followed by hydrolysis. The reaction conditions often include the use of lithium hydroxide monohydrate in an aqueous THF/MeOH solution . This method allows for the formation of the cyclobutylidene group, which is crucial for the compound’s unique properties.
Industrial Production Methods: While specific industrial production methods for this compound are not extensively documented, the general approach involves large-scale organic synthesis techniques. These methods would likely include the use of automated reactors and continuous flow systems to ensure high yield and purity.
Chemical Reactions Analysis
Types of Reactions: 2-Cyclobutylideneacetic acid undergoes various chemical reactions, including:
Oxidation: This reaction can be facilitated by oxidizing agents such as potassium permanganate or chromium trioxide.
Reduction: Reduction reactions typically involve reagents like lithium aluminum hydride or sodium borohydride.
Substitution: Common substitution reactions include halogenation and nitration, often using reagents like halogens (Cl2, Br2) or nitric acid.
Common Reagents and Conditions:
Oxidation: Potassium permanganate in an acidic medium.
Reduction: Lithium aluminum hydride in anhydrous ether.
Substitution: Halogens in the presence of a catalyst like iron(III) chloride.
Major Products Formed:
Oxidation: Cyclobutylideneacetic acid can be converted to cyclobutylideneacetone.
Reduction: The compound can be reduced to cyclobutylideneethanol.
Substitution: Halogenation can yield halocyclobutylideneacetic acids.
Scientific Research Applications
2-Cyclobutylideneacetic acid has a wide range of applications in scientific research:
Chemistry: It is used as a building block in organic synthesis, particularly in the formation of complex cyclic structures.
Biology: The compound is studied for its potential biological activity, including its effects on cellular processes.
Medicine: Research is ongoing to explore its potential as a pharmaceutical intermediate.
Industry: It is used in the synthesis of specialty chemicals and materials.
Mechanism of Action
The mechanism of action of 2-Cyclobutylideneacetic acid involves its interaction with specific molecular targets. The compound can act as an inhibitor or activator of certain enzymes, depending on its structural modifications. The pathways involved often include signal transduction mechanisms and metabolic pathways .
Comparison with Similar Compounds
- Cyclopropylideneacetic acid
- Cyclopentylideneacetic acid
- Cyclohexylideneacetic acid
Comparison: 2-Cyclobutylideneacetic acid is unique due to its four-membered cyclobutylidene ring, which imparts distinct steric and electronic properties. Compared to cyclopropylideneacetic acid, it has a larger ring size, leading to different reactivity and stability. Cyclopentylideneacetic acid, with a five-membered ring, exhibits different conformational dynamics and reactivity patterns .
Properties
IUPAC Name |
2-cyclobutylideneacetic acid | |
---|---|---|
Source | PubChem | |
URL | https://pubchem.ncbi.nlm.nih.gov | |
Description | Data deposited in or computed by PubChem | |
InChI |
InChI=1S/C6H8O2/c7-6(8)4-5-2-1-3-5/h4H,1-3H2,(H,7,8) | |
Source | PubChem | |
URL | https://pubchem.ncbi.nlm.nih.gov | |
Description | Data deposited in or computed by PubChem | |
InChI Key |
ACWUGSRELMTOFJ-UHFFFAOYSA-N | |
Source | PubChem | |
URL | https://pubchem.ncbi.nlm.nih.gov | |
Description | Data deposited in or computed by PubChem | |
Canonical SMILES |
C1CC(=CC(=O)O)C1 | |
Source | PubChem | |
URL | https://pubchem.ncbi.nlm.nih.gov | |
Description | Data deposited in or computed by PubChem | |
Molecular Formula |
C6H8O2 | |
Source | PubChem | |
URL | https://pubchem.ncbi.nlm.nih.gov | |
Description | Data deposited in or computed by PubChem | |
DSSTOX Substance ID |
DTXSID40620160 | |
Record name | Cyclobutylideneacetic acid | |
Source | EPA DSSTox | |
URL | https://comptox.epa.gov/dashboard/DTXSID40620160 | |
Description | DSSTox provides a high quality public chemistry resource for supporting improved predictive toxicology. | |
Molecular Weight |
112.13 g/mol | |
Source | PubChem | |
URL | https://pubchem.ncbi.nlm.nih.gov | |
Description | Data deposited in or computed by PubChem | |
CAS No. |
25021-04-9 | |
Record name | Cyclobutylideneacetic acid | |
Source | EPA DSSTox | |
URL | https://comptox.epa.gov/dashboard/DTXSID40620160 | |
Description | DSSTox provides a high quality public chemistry resource for supporting improved predictive toxicology. | |
Record name | 2-Cyclobutylideneacetic acid | |
Source | European Chemicals Agency (ECHA) | |
URL | https://echa.europa.eu/information-on-chemicals | |
Description | The European Chemicals Agency (ECHA) is an agency of the European Union which is the driving force among regulatory authorities in implementing the EU's groundbreaking chemicals legislation for the benefit of human health and the environment as well as for innovation and competitiveness. | |
Explanation | Use of the information, documents and data from the ECHA website is subject to the terms and conditions of this Legal Notice, and subject to other binding limitations provided for under applicable law, the information, documents and data made available on the ECHA website may be reproduced, distributed and/or used, totally or in part, for non-commercial purposes provided that ECHA is acknowledged as the source: "Source: European Chemicals Agency, http://echa.europa.eu/". Such acknowledgement must be included in each copy of the material. ECHA permits and encourages organisations and individuals to create links to the ECHA website under the following cumulative conditions: Links can only be made to webpages that provide a link to the Legal Notice page. | |
Retrosynthesis Analysis
AI-Powered Synthesis Planning: Our tool employs the Template_relevance Pistachio, Template_relevance Bkms_metabolic, Template_relevance Pistachio_ringbreaker, Template_relevance Reaxys, Template_relevance Reaxys_biocatalysis model, leveraging a vast database of chemical reactions to predict feasible synthetic routes.
One-Step Synthesis Focus: Specifically designed for one-step synthesis, it provides concise and direct routes for your target compounds, streamlining the synthesis process.
Accurate Predictions: Utilizing the extensive PISTACHIO, BKMS_METABOLIC, PISTACHIO_RINGBREAKER, REAXYS, REAXYS_BIOCATALYSIS database, our tool offers high-accuracy predictions, reflecting the latest in chemical research and data.
Strategy Settings
Precursor scoring | Relevance Heuristic |
---|---|
Min. plausibility | 0.01 |
Model | Template_relevance |
Template Set | Pistachio/Bkms_metabolic/Pistachio_ringbreaker/Reaxys/Reaxys_biocatalysis |
Top-N result to add to graph | 6 |
Feasible Synthetic Routes
Disclaimer and Information on In-Vitro Research Products
Please be aware that all articles and product information presented on BenchChem are intended solely for informational purposes. The products available for purchase on BenchChem are specifically designed for in-vitro studies, which are conducted outside of living organisms. In-vitro studies, derived from the Latin term "in glass," involve experiments performed in controlled laboratory settings using cells or tissues. It is important to note that these products are not categorized as medicines or drugs, and they have not received approval from the FDA for the prevention, treatment, or cure of any medical condition, ailment, or disease. We must emphasize that any form of bodily introduction of these products into humans or animals is strictly prohibited by law. It is essential to adhere to these guidelines to ensure compliance with legal and ethical standards in research and experimentation.