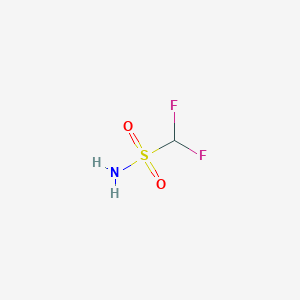
Difluoromethanesulfonamide
Overview
Description
Difluoromethanesulfonamide is a chemical compound characterized by the presence of two fluorine atoms attached to a methanesulfonamide group
Mechanism of Action
Target of Action
Difluoromethanesulfonamide is a novel pharmacophore for the inhibition of an enzyme known as carbonic anhydrase . Carbonic anhydrase plays a crucial role in maintaining pH and fluid balance in the body, making it a significant target for therapeutic interventions .
Mode of Action
The interaction of this compound with carbonic anhydrase results in the inhibition of this enzyme This inhibition disrupts the normal function of the enzyme, leading to changes in pH and fluid balance within the body
Biochemical Pathways
The primary biochemical pathway affected by this compound is the carbonic anhydrase pathway . By inhibiting carbonic anhydrase, this compound disrupts the conversion of carbon dioxide and water into bicarbonate and protons, a reaction that is crucial for maintaining pH balance and fluid balance in various biological systems .
Pharmacokinetics
The compound’s water solubility and stability, as well as its ether partition coefficient and submicromolar dissociation constants for human carbonic anhydrase isozyme ii (hca ii), suggest that it may have promising pharmacokinetic properties . These properties could potentially influence the compound’s bioavailability, although further studies are needed to confirm this.
Result of Action
The molecular and cellular effects of this compound’s action primarily involve the inhibition of carbonic anhydrase . This inhibition can disrupt normal cellular processes that depend on this enzyme, such as pH regulation and fluid balance. The specific cellular effects of this compound may vary depending on the cell type and the presence of different isozymes of carbonic anhydrase.
Action Environment
The action, efficacy, and stability of this compound can be influenced by various environmental factors. For instance, the pH of the environment can affect the compound’s solubility and stability, potentially influencing its ability to interact with its target Additionally, the presence of other substances in the environment, such as other drugs or biological molecules, could potentially interact with this compound and alter its effects
Biochemical Analysis
Biochemical Properties
Difluoromethanesulfonamide plays a crucial role in biochemical reactions, primarily through its interaction with enzymes and proteins. One of the most notable interactions is with carbonic anhydrase isozyme II (HCA II), where this compound acts as an inhibitor . This interaction is characterized by the formation of a stable complex, which effectively inhibits the enzyme’s activity. The compound’s water solubility, stability, and submicromolar dissociation constants make it a promising candidate for therapeutic applications, such as in the treatment of glaucoma .
Cellular Effects
This compound has been shown to influence various cellular processes. It affects cell signaling pathways, gene expression, and cellular metabolism. For instance, its inhibition of carbonic anhydrase can lead to alterations in pH regulation within cells, impacting cellular metabolism and function . Additionally, this compound’s interaction with specific enzymes can modulate gene expression, further influencing cellular activities.
Molecular Mechanism
At the molecular level, this compound exerts its effects through binding interactions with biomolecules. The compound binds to the active site of carbonic anhydrase, inhibiting its enzymatic activity . This inhibition is achieved through the formation of a stable complex between this compound and the enzyme, preventing the enzyme from catalyzing its substrate. This mechanism of action highlights the compound’s potential as a therapeutic agent in conditions where carbonic anhydrase activity needs to be regulated.
Temporal Effects in Laboratory Settings
In laboratory settings, the effects of this compound have been observed to change over time. The compound’s stability and degradation are critical factors that influence its long-term effects on cellular function. Studies have shown that this compound remains stable under various conditions, maintaining its inhibitory activity over extended periods
Dosage Effects in Animal Models
The effects of this compound vary with different dosages in animal models. At lower doses, the compound effectively inhibits carbonic anhydrase without causing significant adverse effects . At higher doses, toxic effects may be observed, including disruptions in cellular metabolism and potential damage to tissues. These dosage-dependent effects highlight the importance of determining optimal dosing regimens for therapeutic applications.
Metabolic Pathways
This compound is involved in various metabolic pathways, primarily through its interaction with enzymes such as carbonic anhydrase . The compound’s inhibition of this enzyme can lead to changes in metabolic flux and metabolite levels, impacting overall cellular metabolism. Additionally, this compound may interact with other enzymes and cofactors, further influencing metabolic pathways.
Transport and Distribution
Within cells and tissues, this compound is transported and distributed through interactions with transporters and binding proteins . These interactions facilitate the compound’s localization and accumulation in specific cellular compartments, where it can exert its inhibitory effects. Understanding the transport and distribution mechanisms of this compound is essential for optimizing its therapeutic potential.
Subcellular Localization
This compound’s subcellular localization is influenced by targeting signals and post-translational modifications . The compound is directed to specific compartments or organelles, where it can interact with its target enzymes. This localization is crucial for the compound’s activity and function, as it ensures that this compound reaches its intended site of action within the cell.
Preparation Methods
Synthetic Routes and Reaction Conditions
The synthesis of difluoromethanesulfonamide typically involves the preparation of key intermediates such as carboxythis compound. One common method involves the reaction of N-protected α-amino acid methyl esters with methanesulfonamide carbanions, followed by electrophilic fluorination . This process yields this compound with high efficiency and without epimerization.
Industrial Production Methods
Industrial production of this compound often employs similar synthetic routes but on a larger scale. The use of efficient fluorination reagents and optimized reaction conditions ensures high yield and purity of the final product .
Chemical Reactions Analysis
Types of Reactions
Difluoromethanesulfonamide undergoes various chemical reactions, including:
Oxidation: The compound can be oxidized under specific conditions to form sulfonyl fluorides.
Reduction: Reduction reactions can convert this compound into corresponding amines.
Substitution: Nucleophilic substitution reactions are common, where the sulfonamide group is replaced by other nucleophiles.
Common Reagents and Conditions
Oxidation: Common oxidizing agents include hydrogen peroxide and peracids.
Reduction: Reducing agents such as lithium aluminum hydride are often used.
Substitution: Nucleophiles like amines and alcohols are employed under basic conditions.
Major Products Formed
The major products formed from these reactions include sulfonyl fluorides, amines, and substituted sulfonamides, depending on the reaction conditions and reagents used .
Scientific Research Applications
Difluoromethanesulfonamide has a wide range of applications in scientific research:
Chemistry: It is used as a reagent in organic synthesis, particularly in the formation of carbon-fluorine bonds.
Comparison with Similar Compounds
Similar Compounds
Trifluoromethanesulfonamide: Similar in structure but with three fluorine atoms, it is used as a strong acid and catalyst in organic synthesis.
Sulfonimidates: These compounds have a sulfur-nitrogen bond and are used as intermediates in the synthesis of other sulfur-containing compounds.
Uniqueness
Difluoromethanesulfonamide is unique due to its specific fluorine substitution pattern, which imparts distinct chemical properties such as high stability and reactivity. Its ability to act as a carbonic anhydrase inhibitor also sets it apart from other sulfonamides .
Properties
IUPAC Name |
difluoromethanesulfonamide | |
---|---|---|
Source | PubChem | |
URL | https://pubchem.ncbi.nlm.nih.gov | |
Description | Data deposited in or computed by PubChem | |
InChI |
InChI=1S/CH3F2NO2S/c2-1(3)7(4,5)6/h1H,(H2,4,5,6) | |
Source | PubChem | |
URL | https://pubchem.ncbi.nlm.nih.gov | |
Description | Data deposited in or computed by PubChem | |
InChI Key |
CWKHJADKQVHTAU-UHFFFAOYSA-N | |
Source | PubChem | |
URL | https://pubchem.ncbi.nlm.nih.gov | |
Description | Data deposited in or computed by PubChem | |
Canonical SMILES |
C(F)(F)S(=O)(=O)N | |
Source | PubChem | |
URL | https://pubchem.ncbi.nlm.nih.gov | |
Description | Data deposited in or computed by PubChem | |
Molecular Formula |
CH3F2NO2S | |
Source | PubChem | |
URL | https://pubchem.ncbi.nlm.nih.gov | |
Description | Data deposited in or computed by PubChem | |
DSSTOX Substance ID |
DTXSID10619561 | |
Record name | 1,1-Difluoromethanesulfonamide | |
Source | EPA DSSTox | |
URL | https://comptox.epa.gov/dashboard/DTXSID10619561 | |
Description | DSSTox provides a high quality public chemistry resource for supporting improved predictive toxicology. | |
Molecular Weight |
131.10 g/mol | |
Source | PubChem | |
URL | https://pubchem.ncbi.nlm.nih.gov | |
Description | Data deposited in or computed by PubChem | |
CAS No. |
50585-74-5 | |
Record name | 1,1-Difluoromethanesulfonamide | |
Source | EPA DSSTox | |
URL | https://comptox.epa.gov/dashboard/DTXSID10619561 | |
Description | DSSTox provides a high quality public chemistry resource for supporting improved predictive toxicology. | |
Retrosynthesis Analysis
AI-Powered Synthesis Planning: Our tool employs the Template_relevance Pistachio, Template_relevance Bkms_metabolic, Template_relevance Pistachio_ringbreaker, Template_relevance Reaxys, Template_relevance Reaxys_biocatalysis model, leveraging a vast database of chemical reactions to predict feasible synthetic routes.
One-Step Synthesis Focus: Specifically designed for one-step synthesis, it provides concise and direct routes for your target compounds, streamlining the synthesis process.
Accurate Predictions: Utilizing the extensive PISTACHIO, BKMS_METABOLIC, PISTACHIO_RINGBREAKER, REAXYS, REAXYS_BIOCATALYSIS database, our tool offers high-accuracy predictions, reflecting the latest in chemical research and data.
Strategy Settings
Precursor scoring | Relevance Heuristic |
---|---|
Min. plausibility | 0.01 |
Model | Template_relevance |
Template Set | Pistachio/Bkms_metabolic/Pistachio_ringbreaker/Reaxys/Reaxys_biocatalysis |
Top-N result to add to graph | 6 |
Feasible Synthetic Routes
Q1: What makes difluoromethanesulfonamide a promising pharmacophore for carbonic anhydrase inhibition, particularly for topical glaucoma therapy?
A1: Difluoromethanesulfonamides exhibit several favorable characteristics for carbonic anhydrase inhibition, especially for topical glaucoma treatment []. Firstly, they demonstrate submicromolar dissociation constants for human carbonic anhydrase isozyme II (HCA II), indicating high binding affinity and potential for potent inhibition []. Secondly, their suitable water solubility and stability profiles, coupled with an appropriate ether partition coefficient, suggest good topical formulation potential and corneal penetration ability [].
Q2: How does the structure of this compound relate to its activity as a steroid sulfatase inhibitor?
A2: A study focusing on steroid sulfatase (STS) inhibition utilized a non-hydrolyzable estrone sulfate analogue modified with a this compound group at the 3-position of the A-ring []. This modification aimed to mimic the sulfate group of estrone sulfate, a natural substrate of STS. The incorporated this compound moiety led to a reversible STS inhibitor with a Ki comparable to its sulfonate analogue at pH 7.0, indicating successful mimicry of the sulfate group []. Interestingly, the difluoro-substituted compound displayed higher potency than its non-fluorinated counterpart, and its inhibitory activity increased with rising pH, a trend opposite to other STS inhibitors []. This suggests that the presence of fluorine atoms in the this compound group significantly influences the interaction with the enzyme and its inhibitory profile.
Q3: What innovative synthetic strategies have been developed for difluoromethanesulfonamides?
A3: Researchers have made significant progress in developing efficient synthetic routes for difluoromethanesulfonamides. One notable approach involves the preparation of carboxythis compound, a versatile intermediate that allows access to a wide variety of this compound derivatives []. This intermediate facilitates the synthesis of compounds like (2-ethanoylamino-1,3,4-thiadiazol-5-yl)-difluoromethanesulfonamide, an analogue of the known carbonic anhydrase inhibitor acetazolamide [].
Q4: Are there specific structural modifications of difluoromethanesulfonamides that influence their herbicidal activity?
A4: Research indicates that specific structural modifications in difluoromethanesulfonanilide derivatives significantly impact their herbicidal activity []. The presence of halogens, variations in the aromatic ring (CH or N), and the nature of substituents (R) on the sulfonamide nitrogen all contribute to their efficacy as herbicides, particularly against paddy field weeds []. These findings suggest that fine-tuning the structure of difluoromethanesulfonamides can lead to the development of compounds with tailored herbicidal properties.
Disclaimer and Information on In-Vitro Research Products
Please be aware that all articles and product information presented on BenchChem are intended solely for informational purposes. The products available for purchase on BenchChem are specifically designed for in-vitro studies, which are conducted outside of living organisms. In-vitro studies, derived from the Latin term "in glass," involve experiments performed in controlled laboratory settings using cells or tissues. It is important to note that these products are not categorized as medicines or drugs, and they have not received approval from the FDA for the prevention, treatment, or cure of any medical condition, ailment, or disease. We must emphasize that any form of bodily introduction of these products into humans or animals is strictly prohibited by law. It is essential to adhere to these guidelines to ensure compliance with legal and ethical standards in research and experimentation.