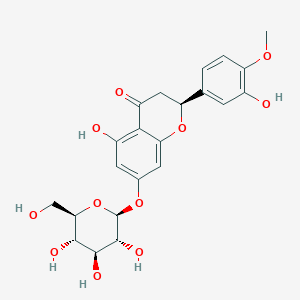
Hesperetin 7-O-glucoside
Overview
Description
Hesperetin 7-O-glucoside is a flavanone glycoside derived from hesperetin, a naturally occurring flavonoid found in citrus fruits. This compound is known for its diverse biological activities, including antioxidant, anti-inflammatory, and antimicrobial properties .
Mechanism of Action
Target of Action
Hesperetin 7-O-glucoside, also known as Eriodictiol-7-glucoside, primarily targets the acyl-coenzyme A:cholesterol acyltransferase genes (ACAT 1 and ACAT 2) and the microsomal triglyceride transfer protein (MTP) activity . These targets play a crucial role in cholesterol and lipid metabolism.
Mode of Action
This compound reduces or inhibits the activity of ACAT 1 and ACAT 2 genes and MTP activity . It also seems to upregulate the LDL receptor . This interaction leads to the reduced assembly and secretion of apoB-containing lipoproteins and enhanced reuptake of those lipoproteins, thereby lowering cholesterol levels .
Biochemical Pathways
The compound affects the cholesterol and lipid metabolism pathways. By inhibiting ACAT 1, ACAT 2, and MTP, it disrupts the normal synthesis and transport of cholesterol and lipids, leading to a decrease in cholesterol levels .
Pharmacokinetics
The oral bioavailability of this compound is influenced by its low water solubility, weak intestinal absorption, disposition via phase II enzymes, and efflux by the enterocytes . Strategies for improving its oral bioavailability include the development of nanoscale drug carriers .
Result of Action
The action of this compound results in a significant reduction in cholesterol levels . This can have beneficial effects in conditions such as hypercholesterolemia and cardiovascular diseases.
Action Environment
The action, efficacy, and stability of this compound can be influenced by various environmental factors. For instance, its solubility can be increased through coordination with metal ions . Additionally, its bioavailability and action can be affected by factors such as temperature, pH, and light .
Biochemical Analysis
Biochemical Properties
Hesperetin 7-O-glucoside plays a crucial role in various biochemical reactions. It interacts with several enzymes, proteins, and other biomolecules. One of the key enzymes involved is flavanone 7-O-glucosyltransferase, which catalyzes the glycosylation of hesperetin at the C-7 position to form this compound . This compound also interacts with intestinal maltase and HMG-CoA reductase, inhibiting their activity . These interactions highlight the compound’s potential in regulating carbohydrate and lipid metabolism.
Cellular Effects
This compound exerts significant effects on various cell types and cellular processes. It has been shown to influence cell signaling pathways, gene expression, and cellular metabolism. For instance, this compound can modulate the production of pro-inflammatory cytokines such as TNF-α and IL-6 in macrophages, thereby exhibiting anti-inflammatory properties . Additionally, it affects the expression of genes involved in oxidative stress response and apoptosis, contributing to its antioxidant and anticancer activities .
Molecular Mechanism
The molecular mechanism of this compound involves several key interactions at the molecular level. It binds to and inhibits the activity of acyl-coenzyme A:cholesterol acyltransferase (ACAT) genes, reducing cholesterol esterification . Furthermore, this compound upregulates the LDL receptor, enhancing the clearance of LDL cholesterol from the bloodstream . These actions contribute to its potential in managing hypercholesterolemia and cardiovascular diseases.
Temporal Effects in Laboratory Settings
In laboratory settings, the effects of this compound have been observed to change over time. The compound is relatively stable under standard laboratory conditions, but its activity can be influenced by factors such as pH and temperature . Long-term studies have shown that this compound can modulate gut microbiota composition and bile acid metabolism in mice, indicating its potential for long-term health benefits .
Dosage Effects in Animal Models
The effects of this compound vary with different dosages in animal models. At lower doses, it exhibits beneficial effects such as anti-inflammatory and antioxidant activities. At higher doses, there may be potential toxic or adverse effects . For example, in a study on mice, dietary administration of this compound at 2.5 and 5 g/kg diet prevented decreases in bone mineral density, but higher doses could lead to adverse effects .
Metabolic Pathways
This compound is involved in several metabolic pathways. It is a precursor for synthesizing neohesperidin dihydrochalcone, a sweetener . The compound is metabolized primarily in the small intestine, where it undergoes hydrolysis to release hesperetin . This process involves enzymes such as rhamnosidase and glucosidase, which facilitate the breakdown and absorption of the compound .
Transport and Distribution
Within cells and tissues, this compound is transported and distributed through various mechanisms. It forms inclusion complexes with β-cyclodextrin, enhancing its solubility and bioavailability . This complexation facilitates its transport across cell membranes and its distribution to target tissues. Additionally, this compound interacts with transporters and binding proteins that aid in its cellular uptake and localization .
Subcellular Localization
This compound is localized in specific subcellular compartments, which influences its activity and function. It is primarily metabolized in the small intestine, particularly in the ileum . The compound’s localization is directed by targeting signals and post-translational modifications that ensure its proper distribution within cells. This subcellular localization is crucial for its biological activities, including its effects on bile acid metabolism and gut microbiota composition .
Preparation Methods
Synthetic Routes and Reaction Conditions: Hesperetin 7-O-glucoside can be synthesized through enzymatic hydrolysis of hesperidin using naringinase enzyme . The process involves the controlled enzymatic hydrolysis of hesperidin, resulting in a high conversion rate of nearly 98% . The reaction conditions are optimized to ensure high purity and yield.
Industrial Production Methods: A novel biosynthesis pathway involves the use of immobilized rhamnosidase on Fe3O4@graphene oxide. This platform facilitates the selective hydrolysis of hesperidin-Cu (II) complex into this compound-Cu (II), which is then dissociated using ammonium hydroxide to obtain high-purity this compound . This method is efficient and scalable for industrial production.
Chemical Reactions Analysis
Types of Reactions: Hesperetin 7-O-glucoside undergoes various chemical reactions, including:
Oxidation: It can be oxidized to form different oxidation products.
Reduction: Reduction reactions can convert it back to hesperetin.
Substitution: Substitution reactions can modify its glycoside moiety.
Common Reagents and Conditions:
Oxidation: Common oxidizing agents include hydrogen peroxide and potassium permanganate.
Reduction: Reducing agents like sodium borohydride are used.
Substitution: Various acids and bases can facilitate substitution reactions.
Major Products:
Oxidation: Oxidized derivatives of hesperetin.
Reduction: Hesperetin.
Substitution: Modified glycoside derivatives.
Scientific Research Applications
Hesperetin 7-O-glucoside has numerous applications in scientific research:
Comparison with Similar Compounds
Hesperidin: A flavanone glycoside found in citrus fruits, known for its low water solubility.
Neohesperidin: A glycoside of hesperetin, used as a sweetener.
Hesperetin: The aglycone form of hesperetin 7-O-glucoside, with potent biological activities.
Uniqueness: this compound stands out due to its higher solubility compared to hesperidin and its efficient conversion to neohesperidin dihydrochalcone
Properties
IUPAC Name |
(2S)-5-hydroxy-2-(3-hydroxy-4-methoxyphenyl)-7-[(2S,3R,4S,5S,6R)-3,4,5-trihydroxy-6-(hydroxymethyl)oxan-2-yl]oxy-2,3-dihydrochromen-4-one | |
---|---|---|
Source | PubChem | |
URL | https://pubchem.ncbi.nlm.nih.gov | |
Description | Data deposited in or computed by PubChem | |
InChI |
InChI=1S/C22H24O11/c1-30-14-3-2-9(4-11(14)24)15-7-13(26)18-12(25)5-10(6-16(18)32-15)31-22-21(29)20(28)19(27)17(8-23)33-22/h2-6,15,17,19-25,27-29H,7-8H2,1H3/t15-,17+,19+,20-,21+,22+/m0/s1 | |
Source | PubChem | |
URL | https://pubchem.ncbi.nlm.nih.gov | |
Description | Data deposited in or computed by PubChem | |
InChI Key |
ADSYMQORONDIDD-ZJHVPRRPSA-N | |
Source | PubChem | |
URL | https://pubchem.ncbi.nlm.nih.gov | |
Description | Data deposited in or computed by PubChem | |
Canonical SMILES |
COC1=C(C=C(C=C1)C2CC(=O)C3=C(C=C(C=C3O2)OC4C(C(C(C(O4)CO)O)O)O)O)O | |
Source | PubChem | |
URL | https://pubchem.ncbi.nlm.nih.gov | |
Description | Data deposited in or computed by PubChem | |
Isomeric SMILES |
COC1=C(C=C(C=C1)[C@@H]2CC(=O)C3=C(C=C(C=C3O2)O[C@H]4[C@@H]([C@H]([C@@H]([C@H](O4)CO)O)O)O)O)O | |
Source | PubChem | |
URL | https://pubchem.ncbi.nlm.nih.gov | |
Description | Data deposited in or computed by PubChem | |
Molecular Formula |
C22H24O11 | |
Source | PubChem | |
URL | https://pubchem.ncbi.nlm.nih.gov | |
Description | Data deposited in or computed by PubChem | |
DSSTOX Substance ID |
DTXSID80953661 | |
Record name | 5-Hydroxy-2-(3-hydroxy-4-methoxyphenyl)-4-oxo-3,4-dihydro-2H-1-benzopyran-7-yl hexopyranoside | |
Source | EPA DSSTox | |
URL | https://comptox.epa.gov/dashboard/DTXSID80953661 | |
Description | DSSTox provides a high quality public chemistry resource for supporting improved predictive toxicology. | |
Molecular Weight |
464.4 g/mol | |
Source | PubChem | |
URL | https://pubchem.ncbi.nlm.nih.gov | |
Description | Data deposited in or computed by PubChem | |
CAS No. |
31712-49-9 | |
Record name | Hesperetin 7-glucoside | |
Source | CAS Common Chemistry | |
URL | https://commonchemistry.cas.org/detail?cas_rn=31712-49-9 | |
Description | CAS Common Chemistry is an open community resource for accessing chemical information. Nearly 500,000 chemical substances from CAS REGISTRY cover areas of community interest, including common and frequently regulated chemicals, and those relevant to high school and undergraduate chemistry classes. This chemical information, curated by our expert scientists, is provided in alignment with our mission as a division of the American Chemical Society. | |
Explanation | The data from CAS Common Chemistry is provided under a CC-BY-NC 4.0 license, unless otherwise stated. | |
Record name | Hesperitin-7-beta-D-glucoside | |
Source | ChemIDplus | |
URL | https://pubchem.ncbi.nlm.nih.gov/substance/?source=chemidplus&sourceid=0031712499 | |
Description | ChemIDplus is a free, web search system that provides access to the structure and nomenclature authority files used for the identification of chemical substances cited in National Library of Medicine (NLM) databases, including the TOXNET system. | |
Record name | 5-Hydroxy-2-(3-hydroxy-4-methoxyphenyl)-4-oxo-3,4-dihydro-2H-1-benzopyran-7-yl hexopyranoside | |
Source | EPA DSSTox | |
URL | https://comptox.epa.gov/dashboard/DTXSID80953661 | |
Description | DSSTox provides a high quality public chemistry resource for supporting improved predictive toxicology. | |
Retrosynthesis Analysis
AI-Powered Synthesis Planning: Our tool employs the Template_relevance Pistachio, Template_relevance Bkms_metabolic, Template_relevance Pistachio_ringbreaker, Template_relevance Reaxys, Template_relevance Reaxys_biocatalysis model, leveraging a vast database of chemical reactions to predict feasible synthetic routes.
One-Step Synthesis Focus: Specifically designed for one-step synthesis, it provides concise and direct routes for your target compounds, streamlining the synthesis process.
Accurate Predictions: Utilizing the extensive PISTACHIO, BKMS_METABOLIC, PISTACHIO_RINGBREAKER, REAXYS, REAXYS_BIOCATALYSIS database, our tool offers high-accuracy predictions, reflecting the latest in chemical research and data.
Strategy Settings
Precursor scoring | Relevance Heuristic |
---|---|
Min. plausibility | 0.01 |
Model | Template_relevance |
Template Set | Pistachio/Bkms_metabolic/Pistachio_ringbreaker/Reaxys/Reaxys_biocatalysis |
Top-N result to add to graph | 6 |
Feasible Synthetic Routes
Disclaimer and Information on In-Vitro Research Products
Please be aware that all articles and product information presented on BenchChem are intended solely for informational purposes. The products available for purchase on BenchChem are specifically designed for in-vitro studies, which are conducted outside of living organisms. In-vitro studies, derived from the Latin term "in glass," involve experiments performed in controlled laboratory settings using cells or tissues. It is important to note that these products are not categorized as medicines or drugs, and they have not received approval from the FDA for the prevention, treatment, or cure of any medical condition, ailment, or disease. We must emphasize that any form of bodily introduction of these products into humans or animals is strictly prohibited by law. It is essential to adhere to these guidelines to ensure compliance with legal and ethical standards in research and experimentation.