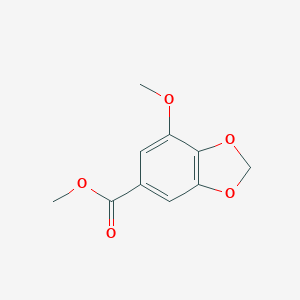
Methyl 7-methoxy-1,3-benzodioxole-5-carboxylate
Overview
Description
Methyl 7-methoxy-1,3-benzodioxole-5-carboxylate is an organic compound with the molecular formula C10H10O5. It is a derivative of benzodioxole, a bicyclic compound consisting of a benzene ring fused with a dioxole ring. This compound is often used as an intermediate in the synthesis of various natural products and pharmaceuticals due to its unique chemical structure and reactivity .
Preparation Methods
Synthetic Routes and Reaction Conditions
The synthesis of Methyl 7-methoxy-1,3-benzodioxole-5-carboxylate typically involves the following steps:
Esterification: Gallic acid is esterified with methanol in the presence of sulfuric acid to form methyl 3,4,5-trihydroxybenzoate.
Methylation: The methyl ester is then methylated using dimethyl sulfate to produce methyl 3-methoxy-4,5-dihydroxybenzoate.
Cyclization: The methylated ester undergoes cyclization with diiodomethane under basic conditions to form methyl 5-methoxy-3,4-methylenedioxybenzoate.
Bromination: Finally, the compound is brominated to yield this compound.
Industrial Production Methods
Industrial production of this compound follows similar synthetic routes but often employs optimized reaction conditions and catalysts to enhance yield and purity. Large-scale production may involve continuous flow reactors and automated systems to ensure consistent quality and efficiency .
Chemical Reactions Analysis
Types of Reactions
Methyl 7-methoxy-1,3-benzodioxole-5-carboxylate undergoes various chemical reactions, including:
Oxidation: The compound can be oxidized to form corresponding carboxylic acids.
Reduction: Reduction reactions can convert the ester group to an alcohol.
Substitution: Electrophilic and nucleophilic substitution reactions can occur on the aromatic ring.
Common Reagents and Conditions
Oxidation: Common oxidizing agents include potassium permanganate and chromium trioxide.
Reduction: Reducing agents such as lithium aluminum hydride or sodium borohydride are used.
Substitution: Reagents like halogens, nitrating agents, and sulfonating agents are employed under controlled conditions.
Major Products
Oxidation: Produces carboxylic acids.
Reduction: Yields alcohols.
Substitution: Forms various substituted benzodioxole derivatives.
Scientific Research Applications
Chemistry
MMB is primarily utilized as an intermediate in the synthesis of complex organic molecules. Its unique structure allows for various chemical reactions, including:
- Esterification : MMB can be synthesized from gallic acid through esterification with methanol.
- Methylation : The compound can undergo methylation to produce derivatives with varying biological activities.
- Cyclization and Bromination : These reactions allow for the formation of more complex structures that can be explored for their biological properties.
Biological Research
MMB has garnered attention for its potential biological activities, particularly in the following areas:
- Antioxidant Activity : Research indicates that MMB possesses antioxidant properties, making it a candidate for drug development targeting oxidative stress-related diseases.
- Anti-inflammatory Properties : Preliminary studies suggest that MMB can modulate inflammatory pathways, indicating its potential utility in treating inflammatory conditions.
- Cytotoxic Effects : In vitro studies have shown that MMB exhibits cytotoxic effects against various cancer cell lines, suggesting its potential role in cancer therapy.
Medicinal Applications
The pharmacological properties of MMB are being explored extensively. Notable findings include:
- Enzyme Inhibition : MMB has been found to inhibit specific enzymes associated with cancer progression, such as EZH1 and EZH2. This highlights its potential role in epigenetic regulation and cancer treatment strategies.
- Therapeutic Potential : Due to its diverse biological activities, MMB is being investigated for potential therapeutic applications across various fields, including oncology and inflammation management.
Industrial Applications
In the industrial sector, MMB is utilized in the production of fine chemicals and pharmaceuticals. Its unique reactivity makes it suitable for synthesizing various compounds used in drug development and chemical manufacturing.
Case Studies
Several case studies have highlighted the biological effects of MMB:
-
Cytotoxicity Against Cancer Cell Lines :
- A study assessed MMB's cytotoxic effects on various cancer cell lines, revealing significant inhibition of cell proliferation at micromolar concentrations. Further investigation into its mechanism could lead to novel anticancer therapies.
-
Anti-inflammatory Effects in Animal Models :
- Research evaluated the anti-inflammatory properties of MMB in rodent models, showing a marked reduction in inflammatory markers following treatment with MMB.
-
Oxidative Stress Modulation :
- Studies focusing on oxidative stress found that MMB effectively reduced oxidative damage in cellular models exposed to reactive oxygen species (ROS), supporting its role as an antioxidant agent.
Mechanism of Action
The mechanism of action of Methyl 7-methoxy-1,3-benzodioxole-5-carboxylate involves its interaction with various molecular targets and pathways. It can inhibit specific enzymes or receptors, leading to alterations in cellular processes. The exact molecular targets and pathways depend on the specific application and context of use.
Comparison with Similar Compounds
Similar Compounds
- Methyl 4-bromo-7-methoxy-1,3-benzodioxole-5-carboxylate
- Methyl 7-(methoxy-d3) benzo[d][1,3]dioxole-5-carboxylate
- 7-Methoxy-1,3-benzodioxole-5-carboxylic acid
Uniqueness
Methyl 7-methoxy-1,3-benzodioxole-5-carboxylate is unique due to its specific substitution pattern on the benzodioxole ring, which imparts distinct chemical and biological properties. This uniqueness makes it valuable in the synthesis of specialized compounds and in research applications .
Biological Activity
Methyl 7-methoxy-1,3-benzodioxole-5-carboxylate (commonly referred to as MMB) is an organic compound with notable biological activities that have attracted significant research interest. This article provides a detailed overview of its biological activity, including mechanisms of action, potential therapeutic applications, and comparative analysis with related compounds.
Chemical Structure and Properties
MMB has a molecular formula of C11H10O5 and a molecular weight of approximately 210.18 g/mol. Its unique structure features a benzodioxole moiety and a methoxy group, contributing to its diverse chemical reactivity and biological properties. The compound can be synthesized through various methods, including oxidation, reduction, and substitution reactions, which are essential for producing derivatives with specific biological activities.
Biological Activities
Research has indicated that MMB exhibits several biological activities:
- Antioxidant Activity : MMB has been identified as a potential lead compound for developing drugs targeting oxidative stress. Its ability to scavenge free radicals may confer protective effects against oxidative damage in cells.
- Anti-inflammatory Properties : Preliminary studies suggest that MMB can modulate inflammatory pathways, making it a candidate for treating inflammatory diseases.
- Cytotoxic Effects : In vitro studies have demonstrated that MMB possesses cytotoxic effects against various cancer cell lines. This suggests its potential utility in cancer therapy, although further studies are needed to clarify its mechanisms of action .
- Enzyme Inhibition : MMB has shown inhibitory action on specific enzymes such as EZH1 and EZH2, which are implicated in cancer progression. This activity highlights its potential role in epigenetic regulation and cancer treatment strategies .
The mechanisms through which MMB exerts its biological effects are multifaceted:
- Interaction with Enzymes : MMB's structure allows it to interact with various enzymes and receptors within biological systems. This interaction can inhibit enzymatic activity or alter signaling pathways, leading to the observed biological effects.
- Cellular Pathway Modulation : By influencing cellular pathways related to inflammation and oxidative stress, MMB may contribute to the overall modulation of cellular responses in pathological conditions.
Comparative Analysis with Related Compounds
To better understand the unique properties of MMB, it is useful to compare it with structurally similar compounds. The following table summarizes key features of MMB and selected analogs:
Compound Name | Molecular Formula | Key Features | Unique Aspects |
---|---|---|---|
Methyl 6-amino-7-methoxy-2H-1,3-benzodioxole-5-carboxylate | C11H12N2O5 | Contains an amino group; different biological profile | Exhibits distinct antimicrobial properties |
Methyl 4,5-dimethoxybenzoate | C10H12O4 | Two methoxy groups on benzene | Lacks the dioxole structure |
Methyl 3-hydroxybenzoate | C8H8O3 | Hydroxy group instead of dioxole | Simpler synthesis route; studied for different activities |
This comparison illustrates how the structural variations impact the biological activities and potential applications of these compounds.
Case Studies
Several case studies have explored the biological effects of MMB:
- Cytotoxicity Against Cancer Cell Lines : One study assessed MMB's cytotoxic effects on various cancer cell lines, revealing significant inhibition of cell proliferation at micromolar concentrations. The study concluded that further investigation into the compound's mechanism could lead to novel anticancer therapies.
- Anti-inflammatory Effects in Animal Models : Another study evaluated the anti-inflammatory properties of MMB in rodent models of inflammation. Results indicated a marked reduction in inflammatory markers following treatment with MMB, suggesting its potential as an anti-inflammatory agent.
- Oxidative Stress Modulation : Research focusing on oxidative stress found that MMB effectively reduced oxidative damage in cellular models exposed to reactive oxygen species (ROS), supporting its role as an antioxidant agent.
Properties
IUPAC Name |
methyl 7-methoxy-1,3-benzodioxole-5-carboxylate | |
---|---|---|
Source | PubChem | |
URL | https://pubchem.ncbi.nlm.nih.gov | |
Description | Data deposited in or computed by PubChem | |
InChI |
InChI=1S/C10H10O5/c1-12-7-3-6(10(11)13-2)4-8-9(7)15-5-14-8/h3-4H,5H2,1-2H3 | |
Source | PubChem | |
URL | https://pubchem.ncbi.nlm.nih.gov | |
Description | Data deposited in or computed by PubChem | |
InChI Key |
DQQKIGXHWZLSCW-UHFFFAOYSA-N | |
Source | PubChem | |
URL | https://pubchem.ncbi.nlm.nih.gov | |
Description | Data deposited in or computed by PubChem | |
Canonical SMILES |
COC1=CC(=CC2=C1OCO2)C(=O)OC | |
Source | PubChem | |
URL | https://pubchem.ncbi.nlm.nih.gov | |
Description | Data deposited in or computed by PubChem | |
Molecular Formula |
C10H10O5 | |
Source | PubChem | |
URL | https://pubchem.ncbi.nlm.nih.gov | |
Description | Data deposited in or computed by PubChem | |
DSSTOX Substance ID |
DTXSID20345086 | |
Record name | Methyl 3-methoxy-4,5-methylenedioxybenzoate | |
Source | EPA DSSTox | |
URL | https://comptox.epa.gov/dashboard/DTXSID20345086 | |
Description | DSSTox provides a high quality public chemistry resource for supporting improved predictive toxicology. | |
Molecular Weight |
210.18 g/mol | |
Source | PubChem | |
URL | https://pubchem.ncbi.nlm.nih.gov | |
Description | Data deposited in or computed by PubChem | |
CAS No. |
22934-58-3 | |
Record name | Methyl 3-methoxy-4,5-methylenedioxybenzoate | |
Source | EPA DSSTox | |
URL | https://comptox.epa.gov/dashboard/DTXSID20345086 | |
Description | DSSTox provides a high quality public chemistry resource for supporting improved predictive toxicology. | |
Synthesis routes and methods I
Procedure details
Synthesis routes and methods II
Procedure details
Synthesis routes and methods III
Procedure details
Synthesis routes and methods IV
Procedure details
Retrosynthesis Analysis
AI-Powered Synthesis Planning: Our tool employs the Template_relevance Pistachio, Template_relevance Bkms_metabolic, Template_relevance Pistachio_ringbreaker, Template_relevance Reaxys, Template_relevance Reaxys_biocatalysis model, leveraging a vast database of chemical reactions to predict feasible synthetic routes.
One-Step Synthesis Focus: Specifically designed for one-step synthesis, it provides concise and direct routes for your target compounds, streamlining the synthesis process.
Accurate Predictions: Utilizing the extensive PISTACHIO, BKMS_METABOLIC, PISTACHIO_RINGBREAKER, REAXYS, REAXYS_BIOCATALYSIS database, our tool offers high-accuracy predictions, reflecting the latest in chemical research and data.
Strategy Settings
Precursor scoring | Relevance Heuristic |
---|---|
Min. plausibility | 0.01 |
Model | Template_relevance |
Template Set | Pistachio/Bkms_metabolic/Pistachio_ringbreaker/Reaxys/Reaxys_biocatalysis |
Top-N result to add to graph | 6 |
Feasible Synthetic Routes
Disclaimer and Information on In-Vitro Research Products
Please be aware that all articles and product information presented on BenchChem are intended solely for informational purposes. The products available for purchase on BenchChem are specifically designed for in-vitro studies, which are conducted outside of living organisms. In-vitro studies, derived from the Latin term "in glass," involve experiments performed in controlled laboratory settings using cells or tissues. It is important to note that these products are not categorized as medicines or drugs, and they have not received approval from the FDA for the prevention, treatment, or cure of any medical condition, ailment, or disease. We must emphasize that any form of bodily introduction of these products into humans or animals is strictly prohibited by law. It is essential to adhere to these guidelines to ensure compliance with legal and ethical standards in research and experimentation.