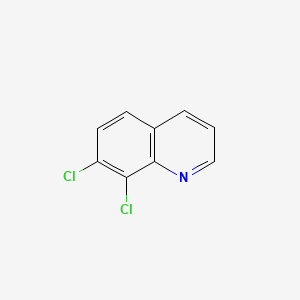
7,8-Dichloroquinoline
Overview
Description
7,8-Dichloroquinoline is a heterocyclic aromatic organic compound with the molecular formula C9H5Cl2N It is a derivative of quinoline, featuring chlorine atoms substituted at the 7th and 8th positions of the quinoline ring
Mechanism of Action
Target of Action
The primary targets of 7,8-Dichloroquinoline are Plasmodium falciparum and cancer cells . Plasmodium falciparum is a protozoan parasite, one of the species of Plasmodium that cause malaria in humans . It is also known to have significant antitumor activity towards three lines of cancer cells, MCF-7 (human breast cancer), HCT-116 (colon carcinoma), and Hela (Cervical carcinoma) cell lines .
Mode of Action
This compound interacts with its targets by inhibiting their growth . In the case of Plasmodium falciparum, it exhibits significant growth inhibition . For cancer cells, it exerts high activity and shows special selectivity toward MCF-7 cells .
Biochemical Pathways
It is known that the compound has a significant impact on the life cycle of plasmodium falciparum, thereby exerting its antimalarial effects . It also affects the growth and proliferation of certain cancer cells .
Pharmacokinetics
It is known that the compound exhibits significant biological activity, suggesting that it has suitable bioavailability .
Result of Action
The action of this compound results in the inhibition of the growth of Plasmodium falciparum, thereby exerting its antimalarial effects . It also inhibits the growth of certain cancer cells, showing significant antitumor activity .
Biochemical Analysis
Biochemical Properties
7,8-Dichloroquinoline plays a significant role in biochemical reactions due to its interaction with various enzymes, proteins, and other biomolecules. It has been observed to interact with cytochrome P450 enzymes, which are involved in the metabolism of many drugs and xenobiotics. The compound can inhibit the activity of these enzymes, leading to altered metabolic pathways. Additionally, this compound has been shown to bind to DNA and RNA, affecting the replication and transcription processes .
Cellular Effects
The effects of this compound on various types of cells and cellular processes are profound. It influences cell function by modulating cell signaling pathways, gene expression, and cellular metabolism. For instance, this compound has been found to inhibit the growth of cancer cells by inducing apoptosis and cell cycle arrest. It also affects the expression of genes involved in cell proliferation and survival . Furthermore, the compound can disrupt cellular metabolism by inhibiting key metabolic enzymes, leading to reduced ATP production and altered metabolic flux .
Molecular Mechanism
At the molecular level, this compound exerts its effects through various mechanisms. It can bind to specific biomolecules, such as enzymes and nucleic acids, altering their structure and function. The compound has been shown to inhibit the activity of topoisomerases, enzymes that are essential for DNA replication and transcription. This inhibition leads to the accumulation of DNA breaks and ultimately cell death . Additionally, this compound can modulate gene expression by interacting with transcription factors and other regulatory proteins .
Temporal Effects in Laboratory Settings
In laboratory settings, the effects of this compound have been observed to change over time. The compound is relatively stable under normal conditions, but it can degrade when exposed to light or high temperatures. Long-term studies have shown that this compound can have lasting effects on cellular function, including persistent inhibition of enzyme activity and sustained changes in gene expression . These temporal effects are important for understanding the compound’s potential therapeutic applications and safety profile.
Dosage Effects in Animal Models
The effects of this compound vary with different dosages in animal models. At low doses, the compound has been shown to have therapeutic effects, such as antimalarial and antimicrobial activity. At higher doses, this compound can exhibit toxic effects, including hepatotoxicity and neurotoxicity . Threshold effects have been observed, where the compound’s efficacy and toxicity are dose-dependent. These findings highlight the importance of careful dosage optimization in the development of this compound-based therapies.
Metabolic Pathways
This compound is involved in various metabolic pathways, interacting with enzymes and cofactors that regulate its metabolism. The compound is primarily metabolized by cytochrome P450 enzymes, which catalyze its oxidation and subsequent conjugation with glucuronic acid or sulfate . These metabolic transformations affect the compound’s bioavailability and excretion. Additionally, this compound can influence metabolic flux by inhibiting key enzymes in glycolysis and the tricarboxylic acid cycle .
Transport and Distribution
The transport and distribution of this compound within cells and tissues are mediated by various transporters and binding proteins. The compound can be actively transported across cell membranes by ATP-binding cassette (ABC) transporters, which play a crucial role in its cellular uptake and efflux . Once inside the cell, this compound can bind to intracellular proteins, affecting its localization and accumulation. These interactions are important for understanding the compound’s pharmacokinetics and pharmacodynamics.
Subcellular Localization
The subcellular localization of this compound is critical for its activity and function. The compound has been found to localize in the nucleus, where it interacts with DNA and RNA, affecting gene expression and replication . Additionally, this compound can accumulate in the mitochondria, disrupting mitochondrial function and leading to altered cellular metabolism . The compound’s localization is influenced by targeting signals and post-translational modifications that direct it to specific cellular compartments.
Preparation Methods
Synthetic Routes and Reaction Conditions: The synthesis of 7,8-Dichloroquinoline typically involves the chlorination of quinoline derivatives. One common method is the reaction of quinoline with chlorine gas in the presence of a catalyst such as iron(III) chloride. The reaction is carried out under controlled conditions to ensure selective chlorination at the desired positions.
Industrial Production Methods: Industrial production of this compound may involve multi-step processes starting from readily available precursors. For example, the chlorination of 4,7-dichloroquinoline can be achieved using phosphorus oxychloride as a chlorinating agent. The process involves hydrolysis, decarboxylation, and subsequent chlorination steps to yield the final product .
Chemical Reactions Analysis
Types of Reactions: 7,8-Dichloroquinoline undergoes various chemical reactions, including:
Nucleophilic Substitution: The chlorine atoms in the quinoline ring can be replaced by nucleophiles such as amines, thiols, or alkoxides.
Oxidation and Reduction: The compound can participate in oxidation-reduction reactions, altering the oxidation state of the nitrogen atom in the quinoline ring.
Coupling Reactions: It can undergo coupling reactions with aryl or alkyl halides to form more complex structures.
Common Reagents and Conditions:
Nucleophilic Substitution: Reagents such as sodium methoxide or primary amines are commonly used under basic conditions.
Oxidation: Oxidizing agents like potassium permanganate or chromium trioxide can be employed.
Reduction: Reducing agents such as lithium aluminum hydride or sodium borohydride are used.
Major Products Formed:
Substitution Products: Depending on the nucleophile, products such as aminoquinolines or alkoxyquinolines are formed.
Oxidation Products: Oxidized derivatives of quinoline.
Reduction Products: Reduced forms of quinoline derivatives.
Scientific Research Applications
7,8-Dichloroquinoline has diverse applications in scientific research:
Medicinal Chemistry: It serves as a precursor for the synthesis of various pharmacologically active compounds, including antimalarial and anticancer agents.
Biological Studies: The compound is used in studies related to enzyme inhibition and receptor binding due to its structural similarity to biologically active molecules.
Material Science: It is explored for its potential use in the development of organic semiconductors and light-emitting diodes (LEDs).
Industrial Applications: It is used in the synthesis of dyes, pigments, and other industrial chemicals.
Comparison with Similar Compounds
4,7-Dichloroquinoline: Another dichloroquinoline derivative used as an intermediate in the synthesis of antimalarial drugs such as chloroquine and hydroxychloroquine.
4-Chloroquinoline: A simpler derivative with applications in the synthesis of various pharmaceuticals.
Uniqueness: 7,8-Dichloroquinoline is unique due to the specific positioning of chlorine atoms, which influences its reactivity and the types of derivatives that can be synthesized. This positional isomerism allows for the exploration of different chemical and biological properties compared to other dichloroquinoline derivatives.
Properties
IUPAC Name |
7,8-dichloroquinoline | |
---|---|---|
Source | PubChem | |
URL | https://pubchem.ncbi.nlm.nih.gov | |
Description | Data deposited in or computed by PubChem | |
InChI |
InChI=1S/C9H5Cl2N/c10-7-4-3-6-2-1-5-12-9(6)8(7)11/h1-5H | |
Source | PubChem | |
URL | https://pubchem.ncbi.nlm.nih.gov | |
Description | Data deposited in or computed by PubChem | |
InChI Key |
BQNIWGUGRICGFJ-UHFFFAOYSA-N | |
Source | PubChem | |
URL | https://pubchem.ncbi.nlm.nih.gov | |
Description | Data deposited in or computed by PubChem | |
Canonical SMILES |
C1=CC2=C(C(=C(C=C2)Cl)Cl)N=C1 | |
Source | PubChem | |
URL | https://pubchem.ncbi.nlm.nih.gov | |
Description | Data deposited in or computed by PubChem | |
Molecular Formula |
C9H5Cl2N | |
Source | PubChem | |
URL | https://pubchem.ncbi.nlm.nih.gov | |
Description | Data deposited in or computed by PubChem | |
DSSTOX Substance ID |
DTXSID00601697 | |
Record name | 7,8-Dichloroquinoline | |
Source | EPA DSSTox | |
URL | https://comptox.epa.gov/dashboard/DTXSID00601697 | |
Description | DSSTox provides a high quality public chemistry resource for supporting improved predictive toxicology. | |
Molecular Weight |
198.05 g/mol | |
Source | PubChem | |
URL | https://pubchem.ncbi.nlm.nih.gov | |
Description | Data deposited in or computed by PubChem | |
CAS No. |
703-49-1 | |
Record name | 7,8-Dichloroquinoline | |
Source | EPA DSSTox | |
URL | https://comptox.epa.gov/dashboard/DTXSID00601697 | |
Description | DSSTox provides a high quality public chemistry resource for supporting improved predictive toxicology. | |
Retrosynthesis Analysis
AI-Powered Synthesis Planning: Our tool employs the Template_relevance Pistachio, Template_relevance Bkms_metabolic, Template_relevance Pistachio_ringbreaker, Template_relevance Reaxys, Template_relevance Reaxys_biocatalysis model, leveraging a vast database of chemical reactions to predict feasible synthetic routes.
One-Step Synthesis Focus: Specifically designed for one-step synthesis, it provides concise and direct routes for your target compounds, streamlining the synthesis process.
Accurate Predictions: Utilizing the extensive PISTACHIO, BKMS_METABOLIC, PISTACHIO_RINGBREAKER, REAXYS, REAXYS_BIOCATALYSIS database, our tool offers high-accuracy predictions, reflecting the latest in chemical research and data.
Strategy Settings
Precursor scoring | Relevance Heuristic |
---|---|
Min. plausibility | 0.01 |
Model | Template_relevance |
Template Set | Pistachio/Bkms_metabolic/Pistachio_ringbreaker/Reaxys/Reaxys_biocatalysis |
Top-N result to add to graph | 6 |
Feasible Synthetic Routes
Q1: How does the positioning of chlorine atoms on the quinoline ring affect antimalarial activity?
A1: The positioning of chlorine atoms on the quinoline ring significantly influences the antimalarial activity of chloroquine-like compounds. [] Research suggests that while a 7-chloro substituent is generally favored for activity, the presence of an additional chlorine atom at the 8-position can either enhance or diminish this activity depending on other structural features of the molecule. This variability highlights the complexity of structure-activity relationships in antimalarial drug development. Further investigation into the specific interactions of these compounds with their target within the parasite, as well as potential impacts on drug accumulation and resistance mechanisms, is crucial for optimizing drug design.
Q2: The research mentions "reversed chloroquine-type compounds". What does this mean and how does it relate to 7,8-dichloroquinoline?
A2: "Reversed chloroquine-type compounds" refers to a strategy in antimalarial drug design where the orientation of the side chain on the quinoline core is altered compared to the classic structure of chloroquine. [] This modification aims to overcome resistance mechanisms developed by the malaria parasite against chloroquine.
Disclaimer and Information on In-Vitro Research Products
Please be aware that all articles and product information presented on BenchChem are intended solely for informational purposes. The products available for purchase on BenchChem are specifically designed for in-vitro studies, which are conducted outside of living organisms. In-vitro studies, derived from the Latin term "in glass," involve experiments performed in controlled laboratory settings using cells or tissues. It is important to note that these products are not categorized as medicines or drugs, and they have not received approval from the FDA for the prevention, treatment, or cure of any medical condition, ailment, or disease. We must emphasize that any form of bodily introduction of these products into humans or animals is strictly prohibited by law. It is essential to adhere to these guidelines to ensure compliance with legal and ethical standards in research and experimentation.