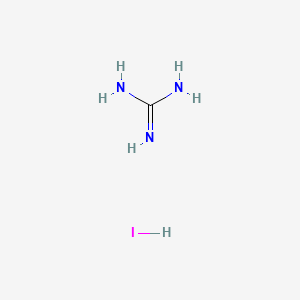
Guanidine Hydroiodide
Overview
Description
Guanidine Hydroiodide is a white crystalline solid that is stable in air. It is soluble in water, methanol, and ethanol, but insoluble in ether and acetone. This compound exhibits strong reducing and basic properties .
Mechanism of Action
Target of Action
Guanidine Hydroiodide primarily targets the neuromuscular junction . It enhances the release of acetylcholine , a neurotransmitter, following a nerve impulse .
Mode of Action
This compound interacts with its targets by enhancing the release of acetylcholine following a nerve impulse . It also appears to slow the rates of depolarization and repolarization of muscle cell membranes .
Biochemical Pathways
It is known that the compound plays a key role in various biological functions . Guanidines serve as valuable scaffolds in organocatalysis and precursors for the synthesis of heterocycles .
Result of Action
The molecular and cellular effects of this compound’s action involve changes in the neuromuscular junction. By enhancing the release of acetylcholine and slowing the rates of depolarization and repolarization of muscle cell membranes, this compound can influence muscle contraction .
Action Environment
This compound is a white crystalline solid that is stable in air . It is soluble in water, methanol, and ethanol, but insoluble in ether and acetone . It has strong reducing and alkaline properties These properties may influence the compound’s action, efficacy, and stability in different environments
Biochemical Analysis
Biochemical Properties
Guanidine Hydroiodide plays a crucial role in biochemical reactions due to its ability to form hydrogen bonds and its high basicity. It interacts with various enzymes, proteins, and other biomolecules. For instance, it has been shown to interact with DNA minor groove binders, kinase inhibitors, and α2-noradrenaline receptors . These interactions are primarily driven by the guanidinium cation’s ability to form multiple resonance structures, which delocalize the positive charge over the entire functional group, enhancing its binding affinity with aromatic systems in biological environments .
Cellular Effects
This compound influences various cellular processes, including cell signaling pathways, gene expression, and cellular metabolism. It has been demonstrated to facilitate cellular translocation and delivery of bioactive cargos through biological barriers . This compound can enhance the cellular uptake of biomolecules by forming charge pairs and hydrogen bonds with cell surface counterparts . Additionally, it has been reported to affect the excitatory postsynaptic potentials in hippocampal neurons, suggesting its role in modulating synaptic activity .
Molecular Mechanism
At the molecular level, this compound exerts its effects through various binding interactions with biomolecules. It acts as a nucleophile in allylation reactions and can form stable guanidinium cations that interact with aromatic systems . These interactions can lead to enzyme inhibition or activation and changes in gene expression. For example, guanidine derivatives have been shown to inhibit certain kinases and modulate the activity of DNA-binding proteins .
Temporal Effects in Laboratory Settings
In laboratory settings, the effects of this compound can change over time. Its stability and degradation are critical factors that influence its long-term effects on cellular function. Studies have shown that guanidine-based compounds can maintain their activity over extended periods, although their efficacy may decrease due to gradual degradation . Long-term exposure to this compound in in vitro and in vivo studies has revealed its potential to induce sustained changes in cellular metabolism and gene expression .
Dosage Effects in Animal Models
The effects of this compound vary with different dosages in animal models. At lower doses, it can enhance synaptic activity and improve cellular uptake of biomolecules . At higher doses, it may exhibit toxic or adverse effects, such as increased excitatory postsynaptic currents and potential neurotoxicity . These threshold effects highlight the importance of careful dosage regulation in experimental settings.
Metabolic Pathways
This compound is involved in various metabolic pathways, interacting with enzymes and cofactors. It can act as a precursor for the synthesis of other guanidine derivatives, which play essential roles in biological functions . The compound’s high basicity allows it to participate in reactions that modify metabolic flux and alter metabolite levels .
Transport and Distribution
Within cells and tissues, this compound is transported and distributed through interactions with specific transporters and binding proteins. Its ability to form hydrogen bonds facilitates its movement across cellular membranes and its accumulation in particular cellular compartments . This transport mechanism is crucial for its biological activity and effectiveness in various applications.
Subcellular Localization
This compound’s subcellular localization is influenced by targeting signals and post-translational modifications. It can be directed to specific compartments or organelles, where it exerts its effects on cellular function . For example, its localization in the nucleus can enhance its interaction with DNA-binding proteins and influence gene expression .
Preparation Methods
Guanidine Hydroiodide can be synthesized through the reaction of guanidine with hydroiodic acid. The reaction typically involves the following steps:
- Dissolving guanidine in water.
- Adding hydroiodic acid to the solution.
- Allowing the mixture to react, forming this compound as a precipitate.
- Filtering and drying the precipitate to obtain the final product .
Industrial production methods often involve similar steps but on a larger scale, with careful control of reaction conditions to ensure high yield and purity.
Chemical Reactions Analysis
Guanidine Hydroiodide undergoes various types of chemical reactions, including:
Oxidation: this compound can be oxidized to form guanidine nitrate.
Reduction: It can be reduced to form guanidine.
Substitution: This compound can undergo substitution reactions with various reagents to form different guanidine derivatives
Common reagents used in these reactions include oxidizing agents like nitric acid, reducing agents like hydrogen gas, and various organic compounds for substitution reactions. The major products formed from these reactions depend on the specific reagents and conditions used.
Scientific Research Applications
Guanidine Hydroiodide has a wide range of applications in scientific research:
Chemistry: It is used as a reagent in organic synthesis and as a catalyst in various chemical reactions.
Biology: this compound is used in the study of protein denaturation and as a chaotropic agent to disrupt hydrogen bonds in proteins and nucleic acids.
Medicine: It has applications in the development of pharmaceuticals, particularly in the synthesis of drugs that target specific biological pathways.
Industry: This compound is used in the production of dyes and pigments, as well as in the manufacture of other chemical intermediates .
Comparison with Similar Compounds
Guanidine Hydroiodide can be compared with other guanidine derivatives such as:
Guanidine Hydrochloride: Similar in structure but with different solubility and reactivity properties.
Guanidine Nitrate: An oxidized form of guanidine with different applications in explosives and propellants.
Thiourea: A sulfur-containing analog of guanidine with different chemical properties and applications
This compound is unique due to its specific solubility profile and strong reducing properties, making it particularly useful in certain chemical and biological applications.
Properties
IUPAC Name |
guanidine;hydroiodide | |
---|---|---|
Source | PubChem | |
URL | https://pubchem.ncbi.nlm.nih.gov | |
Description | Data deposited in or computed by PubChem | |
InChI |
InChI=1S/CH5N3.HI/c2-1(3)4;/h(H5,2,3,4);1H | |
Source | PubChem | |
URL | https://pubchem.ncbi.nlm.nih.gov | |
Description | Data deposited in or computed by PubChem | |
InChI Key |
UUDRLGYROXTISK-UHFFFAOYSA-N | |
Source | PubChem | |
URL | https://pubchem.ncbi.nlm.nih.gov | |
Description | Data deposited in or computed by PubChem | |
Canonical SMILES |
C(=N)(N)N.I | |
Source | PubChem | |
URL | https://pubchem.ncbi.nlm.nih.gov | |
Description | Data deposited in or computed by PubChem | |
Molecular Formula |
CH6IN3 | |
Source | PubChem | |
URL | https://pubchem.ncbi.nlm.nih.gov | |
Description | Data deposited in or computed by PubChem | |
Molecular Weight |
186.98 g/mol | |
Source | PubChem | |
URL | https://pubchem.ncbi.nlm.nih.gov | |
Description | Data deposited in or computed by PubChem | |
CAS No. |
19227-70-4 | |
Record name | Guanidine, monohydriodide | |
Source | CAS Common Chemistry | |
URL | https://commonchemistry.cas.org/detail?cas_rn=19227-70-4 | |
Description | CAS Common Chemistry is an open community resource for accessing chemical information. Nearly 500,000 chemical substances from CAS REGISTRY cover areas of community interest, including common and frequently regulated chemicals, and those relevant to high school and undergraduate chemistry classes. This chemical information, curated by our expert scientists, is provided in alignment with our mission as a division of the American Chemical Society. | |
Explanation | The data from CAS Common Chemistry is provided under a CC-BY-NC 4.0 license, unless otherwise stated. | |
Retrosynthesis Analysis
AI-Powered Synthesis Planning: Our tool employs the Template_relevance Pistachio, Template_relevance Bkms_metabolic, Template_relevance Pistachio_ringbreaker, Template_relevance Reaxys, Template_relevance Reaxys_biocatalysis model, leveraging a vast database of chemical reactions to predict feasible synthetic routes.
One-Step Synthesis Focus: Specifically designed for one-step synthesis, it provides concise and direct routes for your target compounds, streamlining the synthesis process.
Accurate Predictions: Utilizing the extensive PISTACHIO, BKMS_METABOLIC, PISTACHIO_RINGBREAKER, REAXYS, REAXYS_BIOCATALYSIS database, our tool offers high-accuracy predictions, reflecting the latest in chemical research and data.
Strategy Settings
Precursor scoring | Relevance Heuristic |
---|---|
Min. plausibility | 0.01 |
Model | Template_relevance |
Template Set | Pistachio/Bkms_metabolic/Pistachio_ringbreaker/Reaxys/Reaxys_biocatalysis |
Top-N result to add to graph | 6 |
Feasible Synthetic Routes
Q1: What are the key applications of Guanidine Hydroiodide in material science?
A1: this compound (GAI) has shown promise in the development of organic-inorganic hybrid perovskite materials for solar cell applications. [, , ] Research indicates that GAI can be used as a precursor in the fabrication of thin films for these solar cells. [, , ] Specifically, GAI reacts with tin iodide (SnI2) to form the perovskite structure C(NH2)3SnI3. []
Q2: How does the addition of this compound impact perovskite solar cell performance?
A2: Studies have explored the impact of adding this compound (GAI) and related compounds during different stages of perovskite solar cell fabrication. [] These studies suggest that GAI, when added to specific precursor solutions, can influence the crystallinity and orientation of the perovskite structure, ultimately impacting solar cell efficiency and stability. [] The exact mechanism of this impact varies depending on the stage at which GAI is introduced during the synthesis process. []
Q3: Are there insights into the reaction dynamics of this compound with Tin Iodide in thin film formation?
A3: Yes, in situ infrared multiple-angle incidence-resolved spectroscopy (IR-MAIRS) analysis has been used to study the formation of C(NH2)3SnI3 (GASnI3) thin films from GAI and SnI2 bilayers. [] This research suggests a diffusion-controlled reaction mechanism, where the formation rate of GASnI3 is influenced by the reaction temperature. []
Q4: How does this compound compare to other additives in terms of its impact on perovskite solar cell properties?
A4: Studies have compared the effects of GAI with additives like guanidine thiocyanate (GASCN) and methylamine thiocyanate (MASCN) on perovskite solar cells. [] While all three additives improved cell efficiency and stability, their mechanisms of action differed. [] This suggests that the choice of additive and its integration method can be tailored to target specific improvements in perovskite solar cell performance.
Q5: Beyond solar cells, are there other applications of this compound?
A5: this compound has been investigated for its potential use as a radiopaque substance in medical imaging. [] The presence of iodine in GAI contributes to its ability to absorb X-rays, making it a potential candidate for enhancing the visibility of specific tissues or structures during X-ray examination. []
Q6: Can this compound be synthesized easily?
A6: Yes, one-step synthesis methods for cyclic Guanidine Hydroiodides have been reported. [] These methods involve reacting isothiouronium iodides with various amines in tetrahydrofuran, resulting in the formation of cyclic Guanidine Hydroiodides. [] Subsequent neutralization yields the desired substituted cyclic guanidines. []
Disclaimer and Information on In-Vitro Research Products
Please be aware that all articles and product information presented on BenchChem are intended solely for informational purposes. The products available for purchase on BenchChem are specifically designed for in-vitro studies, which are conducted outside of living organisms. In-vitro studies, derived from the Latin term "in glass," involve experiments performed in controlled laboratory settings using cells or tissues. It is important to note that these products are not categorized as medicines or drugs, and they have not received approval from the FDA for the prevention, treatment, or cure of any medical condition, ailment, or disease. We must emphasize that any form of bodily introduction of these products into humans or animals is strictly prohibited by law. It is essential to adhere to these guidelines to ensure compliance with legal and ethical standards in research and experimentation.