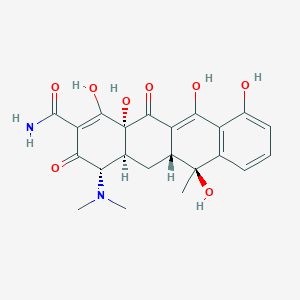
Tetracycline,(S)
- Click on QUICK INQUIRY to receive a quote from our team of experts.
- With the quality product at a COMPETITIVE price, you can focus more on your research.
Overview
Description
Tetracycline (S) refers to a structural subclass of tetracyclines characterized by a specific polyketide folding mechanism (Type S) produced by Streptomyces species. This classification distinguishes it from fungal-derived analogs like viridicatumtoxin (Type F) . Tetracycline (S) is a broad-spectrum antibiotic inhibiting bacterial protein synthesis by binding to the 30S ribosomal subunit.
Preparation Methods
Tetracycline can be synthesized through various methods. One common method involves the fermentation of specific strains of Streptomyces bacteria, followed by chemical modification . Industrial production methods often involve large-scale fermentation processes, followed by purification and chemical modification to obtain the final product .
Chemical Reactions Analysis
Chelation and Metal Complexation
Tetracycline forms stable complexes with divalent and trivalent metal ions (e.g., Mg²⁺, Fe²⁺, Fe³⁺, Ca²⁺) via its β-diketone system (C11–C12), enolic (C1–C3), and carboxamide (C2) groups . This chelation modulates its antimicrobial efficacy and environmental reactivity:
-
Fe(II) Complexation : Accelerates Fe(II) oxidation to Fe(III) in the presence of oxygen, generating reactive oxygen species (ROS) like ·OH, which degrade tetracycline .
-
Mg²⁺ Binding : Stabilizes tetracycline’s interaction with the bacterial ribosome by coordinating with the keto-enol system .
Enzymatic Oxidation and Degradation
Tetracycline-inactivating enzymes (e.g., TetX) catalyze flavin-dependent monooxygenation, primarily hydroxylating C11a or C12a . This disrupts Mg²⁺ chelation and ribosome binding. Key findings include:
-
TetX Mechanism : Uses NADPH to reduce FAD, forming a C4a-peroxyflavin intermediate that transfers an electrophilic hydroxyl group to tetracycline .
-
Degradation Products :
Acid-Catalyzed Dehydration
Under acidic conditions, tetracycline undergoes dehydration at C6–C5a, forming anhydrotetracycline . This product lacks antibacterial activity due to structural flattening (Figure 11) .
Alkaline Hydrolysis
Degrades via cleavage of the C11–C12 β-diketone system, yielding isotetracycline and other fragments .
Redox Reactions with Iron
Tetracycline participates in iron redox cycling:
-
Fe(II) Oxidation :
Fe(II)–TC+O2→Fe(III)–TC+\cdotpOH -
Fe(III) Reduction :
Fe(III)–TC complexes slowly reduce to Fe(II), perpetuating redox cycles even under anaerobic conditions .
Structural Dynamics and Tautomerism
Tetracycline exists in multiple tautomeric and ionic states (neutral, zwitterionic, cationic, di-anionic), influencing reactivity :
Form | C1–O (Å) | C3–O (Å) | C4–O (Å) | C13–O (Å) |
---|---|---|---|---|
Neutral | 1.272 | 1.231 | 1.339 | 1.305 |
Zwitterionic | 1.255 | 1.238 | 1.334 | 1.245 |
Di-anionic | 1.236 | 1.261 | 1.258 | 1.271 |
Data from X-ray crystallography studies .
Environmental Transformation
In aquatic systems, tetracycline degrades via:
Scientific Research Applications
Tetracyclines are broad-spectrum antibiotics widely used since the 1950s to treat various bacterial infections . They are effective against Gram-positive and Gram-negative bacteria, intracellular chlamydiae, mycoplasmas, rickettsiae, and protozoan parasites .
Scientific Research Applications
Antimicrobial Properties
Tetracyclines are valued for their broad-spectrum antimicrobial activity . They inhibit bacterial protein synthesis by binding to the 30S ribosomal subunit, specifically at the Tet-1 site, which interferes with the attachment of aminoacyl-tRNA to the ribosomal A-site . This mechanism effectively halts bacterial growth by preventing the addition of new amino acids to the growing polypeptide chain .
Clinical and Medical Applications
- Treatment of Infections Tetracyclines are used to treat various infections, including those caused by bacteria that could be used in biological weapons . Doxycycline, a tetracycline derivative, can kill Staphylococcus aureus biofilms in prosthetic hip and knee infections . A combination of minocycline and vancomycin has been used for treating chronic methicillin-resistant coagulase-negative staphylococcal prosthetic joint infections . Additionally, a combination of rifampicin and doxycycline has successfully treated multidrug-resistant Acinetobacter infections following hip exchange .
- Wound Healing Tetracycline, when combined with biomaterials, can promote wound healing due to its cytocompatibility and anti-inflammatory effects .
- Prophylaxis Against STIs Doxycycline has been shown to significantly reduce bacterial sexually transmitted infections (STIs) .
Applications in Tissue Engineering
Tetracycline is being explored as a component in cellular scaffolds for tissue engineering due to its biocompatibility and broad-spectrum antimicrobial capacity . It can be used as a drug delivery system, potentially reducing the risk of antibiotic resistance through targeted application and reduced dosage .
Veterinary Medicine
Tetracycline is also used in veterinary medicine. However, the prevalence of antimicrobial-resistant Campylobacter is high in both antimicrobial-free and conventional pig production systems, with the highest resistance observed against tetracycline .
Case Studies
- Successful Seromadesis with Doxycycline : A 69-year-old man with a methicillin-sensitive Staphylococcus aureus prosthetic hip infection and a recurrent seroma cavity was successfully treated by injecting 200 mg of doxycycline into the cavity, leading to seromadesis .
- Treatment of Brucella Infections : Several cases of periprosthetic hip and knee infections caused by Brucella species were successfully treated using a combination of rifampicin and doxycycline, often involving a two-stage exchange arthroplasty . For example, a 78-year-old man with a prosthetic knee infection caused by Brucella was effectively treated with a two-stage revision arthroplasty and an 8-week course of oral rifampicin and doxycycline .
- Efficacy in Joint Arthroplasties : Clinical studies support the use of tetracyclines in treating infections related to arthroplasty .
Antimicrobial Resistance
Mechanism of Action
Tetracycline exerts its effects by binding to the bacterial 30S ribosomal subunit, preventing the binding of aminoacyl-tRNA to the mRNA-ribosome complex . This inhibition of protein synthesis leads to a bacteriostatic effect, meaning it inhibits the growth of bacteria rather than killing them . Tetracycline can also bind to the bacterial 50S ribosomal subunit and alter the cytoplasmic membrane, causing intracellular components to leak from bacterial cells .
Comparison with Similar Compounds
Comparison with Similar Compounds
Pharmacokinetic Profiles
Bioavailability varies significantly among tetracycline derivatives, particularly between free bases and hydrochloride salts:
Compound | Formulation | Bioavailability (%) | Key Findings |
---|---|---|---|
Chlortetracycline | Free base | 55–60 | Lower solubility, delayed absorption |
Chlortetracycline | Hydrochloride | 75–80 | Enhanced dissolution and rapid peak plasma levels |
Methacycline | Free base | 65–70 | Moderate absorption, pH-dependent stability |
Methacycline | Hydrochloride | 85–90 | Superior gastrointestinal absorption |
Hydrochloride salts generally improve solubility and absorption, making them clinically preferred .
Antimicrobial Efficacy
Comparative studies reveal differences in minimal inhibitory concentrations (MICs) against common pathogens:
Semisynthetic derivatives and novel compounds (e.g., Compound 1 from Pseudonocardia) often exhibit superior potency, suggesting evolutionary optimization for specific bacterial targets .
Non-Antimicrobial Therapeutic Potential
Tetracycline (S) exhibits broader therapeutic applications compared to structural analogs:
Application | Tetracycline (S) Efficacy | Comparable Compounds |
---|---|---|
Rosacea | Anti-inflammatory MMP-9 inhibition | Doxycycline (moderate MMP-9 suppression) |
Cancer Cell Inhibition | Apoptosis induction via mitochondrial pathways | No direct analogs reported |
Neuroprotection | Reduces α-synuclein aggregation in Parkinson’s models | None identified |
These pleiotropic effects are attributed to its chelation properties and modulation of cellular signaling pathways, largely unexplored in other tetracyclines .
Q & A
Basic Research Questions
Q. How should researchers standardize tetracycline concentrations in amphibian biomarker studies to avoid false negatives?
Methodological Answer:
- Concentration Optimization : Prior studies in frogs (e.g., Rana spp.) showed no detectable fluorescence at 250–500 mg/L tetracycline after 24-hour immersion. Validate concentrations using dose-response assays, adjusting exposure duration (e.g., 48–72 hours) and route (oral vs. injection) .
- Validation Controls : Include UV microscopy controls for autofluorescence in untreated tissues and spiked samples with known tetracycline residues. Use histological sections ≤5 µm thick to enhance detection sensitivity .
Q. What are essential controls for tetracycline-based bacterial inhibition assays in antimicrobial resistance (AMR) studies?
Methodological Answer:
- Positive/Negative Controls : Use Escherichia coli ATCC 25922 (tetracycline-sensitive) and Staphylococcus aureus with known tet(M) efflux genes as controls. Include solvent-only controls to rule out vehicle interference (e.g., DMSO) .
- Reference Standards : Calibrate MIC (Minimum Inhibitory Concentration) assays with USP-grade tetracycline hydrochloride (CAS 64-75-5) stored at -20°C to prevent degradation .
Advanced Research Questions
Q. How can researchers resolve contradictions in tetracycline resistance mechanisms across bacterial species?
Methodological Answer:
- Mechanistic Differentiation : Use transcriptome sequencing (RNA-seq) to distinguish between efflux pumps (e.g., tetA, tetB) and ribosomal protection proteins (e.g., tetM). For example, Lactiplantibacillus plantarum FZJTZ19M1 upregulated ABC transporters under tetracycline stress, while mutations in 16S rRNA were dominant in Shigella dysenteriae .
- Comparative Genomics : Perform BLAST alignment of resistance genes against databases like CARD (Comprehensive Antibiotic Resistance Database) to identify species-specific variants .
Q. What methodological considerations are critical for transcriptome analysis of tetracycline-resistant bacteria?
Methodological Answer:
-
RNA-Seq Workflow :
Step Parameter Requirement RNA Extraction RIN (RNA Integrity Number) ≥8.0 Library Prep rRNA Depletion Use Ribo-Zero kits Sequencing Depth Coverage ≥20 million reads/sample
Q. How can researchers detect tetracycline epimers in soil matrices with high accuracy?
Methodological Answer:
-
UHPLC-MS/MS Protocol :
Parameter Setting Column C18 (2.1 × 100 mm, 1.7 µm) Mobile Phase 0.1% Formic Acid (A) / Acetonitrile (B) Gradient 5% B → 95% B over 10 min Ionization ESI+ (m/z 445→154 for tetracycline)
Q. How should researchers address false negatives in tetracycline biomarker studies for ecological tracking?
Methodological Answer:
Properties
Molecular Formula |
C22H24N2O8 |
---|---|
Molecular Weight |
444.4 g/mol |
IUPAC Name |
(4S,4aS,5aR,6S,12aR)-4-(dimethylamino)-1,6,10,11,12a-pentahydroxy-6-methyl-3,12-dioxo-4,4a,5,5a-tetrahydrotetracene-2-carboxamide |
InChI |
InChI=1S/C22H24N2O8/c1-21(31)8-5-4-6-11(25)12(8)16(26)13-9(21)7-10-15(24(2)3)17(27)14(20(23)30)19(29)22(10,32)18(13)28/h4-6,9-10,15,25-26,29,31-32H,7H2,1-3H3,(H2,23,30)/t9-,10+,15+,21-,22+/m1/s1 |
InChI Key |
NWXMGUDVXFXRIG-IRJIAJLHSA-N |
Isomeric SMILES |
C[C@@]1([C@@H]2C[C@H]3[C@@H](C(=O)C(=C([C@]3(C(=O)C2=C(C4=C1C=CC=C4O)O)O)O)C(=O)N)N(C)C)O |
Canonical SMILES |
CC1(C2CC3C(C(=O)C(=C(C3(C(=O)C2=C(C4=C1C=CC=C4O)O)O)O)C(=O)N)N(C)C)O |
Origin of Product |
United States |
Disclaimer and Information on In-Vitro Research Products
Please be aware that all articles and product information presented on BenchChem are intended solely for informational purposes. The products available for purchase on BenchChem are specifically designed for in-vitro studies, which are conducted outside of living organisms. In-vitro studies, derived from the Latin term "in glass," involve experiments performed in controlled laboratory settings using cells or tissues. It is important to note that these products are not categorized as medicines or drugs, and they have not received approval from the FDA for the prevention, treatment, or cure of any medical condition, ailment, or disease. We must emphasize that any form of bodily introduction of these products into humans or animals is strictly prohibited by law. It is essential to adhere to these guidelines to ensure compliance with legal and ethical standards in research and experimentation.