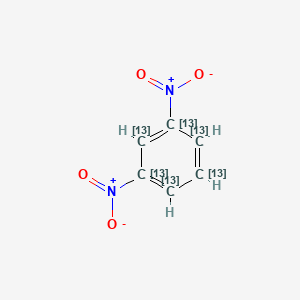
1,3-Dinitrobenzene-13C6
Overview
Description
1,3-Dinitrobenzene-13C6 is a labeled isotopic compound of 1,3-Dinitrobenzene, where all six carbon atoms in the benzene ring are replaced with carbon-13 isotopes. This compound is primarily used in scientific research for tracing and analytical purposes due to its unique isotopic signature. The chemical formula for this compound is 13C6H4(NO2)2, and it has a molecular weight of 174.06 g/mol .
Mechanism of Action
Target of Action
The primary target of 1,3-Dinitrobenzene-13C6 is the enzyme Mannan endo-1,4-beta-mannosidase . This enzyme plays a crucial role in the hydrolysis of beta-1,4-linked mannan and galactomannan .
Mode of Action
It is known that the compound interacts with its target enzyme, potentially altering its function
Action Environment
It is known that the compound is a synthetic chemical used in the manufacture of explosives , suggesting that it may be stable under a variety of conditions.
Biochemical Analysis
Biochemical Properties
1,3-Dinitrobenzene-13C6 plays a crucial role in biochemical reactions due to its interaction with various enzymes and proteins. One of the primary interactions is with the pyruvate dehydrogenase complex (PDHc), where this compound inhibits the complex, leading to metabolic impairment . This inhibition is associated with the loss of lipoic acid immunoreactivity, suggesting that this compound modifies lipoic acid, a cofactor essential for PDHc activity . Additionally, the compound interacts with other biomolecules, inducing oxidative stress and producing reactive intermediates that further affect cellular metabolism .
Cellular Effects
This compound has profound effects on various cell types and cellular processes. In astroglial cells, exposure to this compound results in altered morphology and biochemical dysfunction, consistent with disrupted oxidative energy metabolism . The compound inhibits the pyruvate dehydrogenase complex, leading to decreased cell viability and increased oxidative stress . These effects extend to other cell types, influencing cell signaling pathways, gene expression, and cellular metabolism.
Molecular Mechanism
The molecular mechanism of this compound involves its interaction with the pyruvate dehydrogenase complex. The compound inhibits the complex by modifying lipoic acid, a crucial cofactor for the enzyme’s activity . This inhibition disrupts oxidative energy metabolism, leading to metabolic impairment and increased oxidative stress . Additionally, this compound induces the production of reactive intermediates, further exacerbating cellular dysfunction .
Temporal Effects in Laboratory Settings
In laboratory settings, the effects of this compound change over time. The compound exhibits significant exothermic properties in a closed system, posing a severe risk of explosion . Over time, this compound undergoes thermal decomposition, especially when exposed to mixed acids and other chemicals . These temporal changes affect the compound’s stability and long-term effects on cellular function, as observed in both in vitro and in vivo studies .
Dosage Effects in Animal Models
The effects of this compound vary with different dosages in animal models. At lower doses, the compound induces metabolic impairment and oxidative stress . At higher doses, this compound exhibits toxic effects, including damage to the reproductive and nervous systems . These adverse effects highlight the importance of dosage control in experimental settings to avoid severe toxicity.
Metabolic Pathways
This compound is involved in metabolic pathways that include the inhibition of the pyruvate dehydrogenase complex . This inhibition disrupts the normal metabolic flux, leading to decreased energy production and increased oxidative stress . The compound’s interaction with lipoic acid and other cofactors further affects metabolite levels and overall cellular metabolism .
Transport and Distribution
Within cells and tissues, this compound is transported and distributed through various mechanisms. The compound interacts with transporters and binding proteins that facilitate its localization and accumulation in specific cellular compartments . These interactions influence the compound’s effects on cellular function and its overall distribution within the organism .
Subcellular Localization
This compound localizes to specific subcellular compartments, where it exerts its biochemical effects. The compound’s targeting signals and post-translational modifications direct it to organelles such as mitochondria and the cytoplasm . This subcellular localization is crucial for its activity and function, as it allows this compound to interact with key biomolecules and enzymes within these compartments .
Preparation Methods
1,3-Dinitrobenzene-13C6 is synthesized through the nitration of benzene-13C6. The nitration process involves the reaction of benzene-13C6 with a nitrating mixture, typically composed of concentrated sulfuric acid and nitric acid. The reaction conditions are carefully controlled to ensure the selective formation of the 1,3-dinitro isomer. The reaction proceeds as follows: [ \text{C}_6\text{H}_6 + 2\text{HNO}_3 \rightarrow \text{C}_6\text{H}_4(\text{NO}_2)_2 + 2\text{H}_2\text{O} ]
In industrial settings, the production of this compound follows similar synthetic routes but on a larger scale, with additional purification steps to ensure the high isotopic purity of the final product .
Chemical Reactions Analysis
1,3-Dinitrobenzene-13C6 undergoes various chemical reactions, including:
Reduction: Reduction with sodium sulfide in aqueous solution leads to the formation of 3-nitroaniline.
Common reagents used in these reactions include sodium sulfide, iron, hydrochloric acid, and nitronium tetrafluoroborate. The major products formed are 3-nitroaniline, m-phenylenediamine, and 1,3,5-trinitrobenzene .
Scientific Research Applications
1,3-Dinitrobenzene-13C6 is widely used in scientific research due to its isotopic labeling. Its applications include:
Environmental Analysis: Used as a tracer to study the environmental fate and transport of nitroaromatic compounds.
Synthetic Intermediates: Employed in the synthesis of other isotopically labeled compounds for research purposes.
Explosives Research: Utilized in the study of explosive materials and their degradation products.
Comparison with Similar Compounds
1,3-Dinitrobenzene-13C6 can be compared with other similar compounds, such as:
1,3-Dinitrobenzene: The non-labeled version, which has similar chemical properties but lacks the isotopic signature.
1,3-Dinitrobenzene-15N2: A compound where the nitrogen atoms are replaced with nitrogen-15 isotopes.
1,3-Dinitrobenzene-d4: A deuterated version where hydrogen atoms are replaced with deuterium.
The uniqueness of this compound lies in its carbon-13 labeling, which makes it particularly useful for tracing and analytical studies in various scientific fields .
Properties
IUPAC Name |
1,3-dinitro(1,2,3,4,5,6-13C6)cyclohexa-1,3,5-triene | |
---|---|---|
Source | PubChem | |
URL | https://pubchem.ncbi.nlm.nih.gov | |
Description | Data deposited in or computed by PubChem | |
InChI |
InChI=1S/C6H4N2O4/c9-7(10)5-2-1-3-6(4-5)8(11)12/h1-4H/i1+1,2+1,3+1,4+1,5+1,6+1 | |
Source | PubChem | |
URL | https://pubchem.ncbi.nlm.nih.gov | |
Description | Data deposited in or computed by PubChem | |
InChI Key |
WDCYWAQPCXBPJA-IDEBNGHGSA-N | |
Source | PubChem | |
URL | https://pubchem.ncbi.nlm.nih.gov | |
Description | Data deposited in or computed by PubChem | |
Canonical SMILES |
C1=CC(=CC(=C1)[N+](=O)[O-])[N+](=O)[O-] | |
Source | PubChem | |
URL | https://pubchem.ncbi.nlm.nih.gov | |
Description | Data deposited in or computed by PubChem | |
Isomeric SMILES |
[13CH]1=[13CH][13C](=[13CH][13C](=[13CH]1)[N+](=O)[O-])[N+](=O)[O-] | |
Source | PubChem | |
URL | https://pubchem.ncbi.nlm.nih.gov | |
Description | Data deposited in or computed by PubChem | |
Molecular Formula |
C6H4N2O4 | |
Source | PubChem | |
URL | https://pubchem.ncbi.nlm.nih.gov | |
Description | Data deposited in or computed by PubChem | |
DSSTOX Substance ID |
DTXSID10583995 | |
Record name | 1,3-Dinitro(~13~C_6_)benzene | |
Source | EPA DSSTox | |
URL | https://comptox.epa.gov/dashboard/DTXSID10583995 | |
Description | DSSTox provides a high quality public chemistry resource for supporting improved predictive toxicology. | |
Molecular Weight |
174.06 g/mol | |
Source | PubChem | |
URL | https://pubchem.ncbi.nlm.nih.gov | |
Description | Data deposited in or computed by PubChem | |
CAS No. |
201595-60-0 | |
Record name | 1,3-Dinitro(~13~C_6_)benzene | |
Source | EPA DSSTox | |
URL | https://comptox.epa.gov/dashboard/DTXSID10583995 | |
Description | DSSTox provides a high quality public chemistry resource for supporting improved predictive toxicology. | |
Record name | 201595-60-0 | |
Source | European Chemicals Agency (ECHA) | |
URL | https://echa.europa.eu/information-on-chemicals | |
Description | The European Chemicals Agency (ECHA) is an agency of the European Union which is the driving force among regulatory authorities in implementing the EU's groundbreaking chemicals legislation for the benefit of human health and the environment as well as for innovation and competitiveness. | |
Explanation | Use of the information, documents and data from the ECHA website is subject to the terms and conditions of this Legal Notice, and subject to other binding limitations provided for under applicable law, the information, documents and data made available on the ECHA website may be reproduced, distributed and/or used, totally or in part, for non-commercial purposes provided that ECHA is acknowledged as the source: "Source: European Chemicals Agency, http://echa.europa.eu/". Such acknowledgement must be included in each copy of the material. ECHA permits and encourages organisations and individuals to create links to the ECHA website under the following cumulative conditions: Links can only be made to webpages that provide a link to the Legal Notice page. | |
Disclaimer and Information on In-Vitro Research Products
Please be aware that all articles and product information presented on BenchChem are intended solely for informational purposes. The products available for purchase on BenchChem are specifically designed for in-vitro studies, which are conducted outside of living organisms. In-vitro studies, derived from the Latin term "in glass," involve experiments performed in controlled laboratory settings using cells or tissues. It is important to note that these products are not categorized as medicines or drugs, and they have not received approval from the FDA for the prevention, treatment, or cure of any medical condition, ailment, or disease. We must emphasize that any form of bodily introduction of these products into humans or animals is strictly prohibited by law. It is essential to adhere to these guidelines to ensure compliance with legal and ethical standards in research and experimentation.