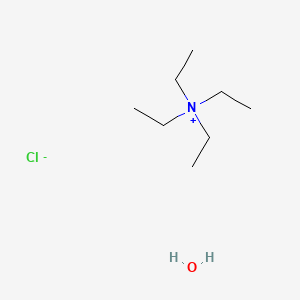
Tetraethylammonium chloride hydrate
Overview
Description
Tetraethylammonium chloride hydrate is a quaternary ammonium compound with the chemical formula ((C_2H_5)_4NCl \cdot xH_2O). It is a hygroscopic, colorless, crystalline solid that exists in two stable hydrates: the monohydrate and the tetrahydrate . This compound is widely used in various scientific research fields due to its unique properties and applications.
Biochemical Analysis
Biochemical Properties
Tetraethylammonium chloride hydrate plays a significant role in biochemical reactions, particularly in the inhibition of potassium channels. It physically enters the pore of the potassium channel and blocks it, thereby affecting the flow of potassium ions. This compound interacts with various enzymes, proteins, and other biomolecules, including choline and acetylcholine. This compound can either mimic or antagonize the actions of these quaternary ammonium ions, influencing their biochemical pathways .
Cellular Effects
This compound has notable effects on various types of cells and cellular processes. It influences cell function by blocking potassium channels, which can affect cell signaling pathways, gene expression, and cellular metabolism. The inhibition of potassium channels by this compound can lead to changes in membrane potential and ion homeostasis, impacting cellular activities such as muscle contraction, neurotransmitter release, and hormone secretion .
Molecular Mechanism
The mechanism of action of this compound involves its binding interactions with potassium channels. By physically entering the pore of the channel, it blocks the passage of potassium ions, leading to the inhibition of potassium channel activity. This compound can also influence enzyme activity by either inhibiting or activating specific enzymes, resulting in changes in gene expression and cellular responses .
Temporal Effects in Laboratory Settings
In laboratory settings, the effects of this compound can change over time. The stability and degradation of this compound are important factors to consider, as they can influence its long-term effects on cellular function. Studies have shown that this compound remains stable under standard laboratory conditions, but its efficacy may decrease over extended periods due to potential degradation .
Dosage Effects in Animal Models
The effects of this compound vary with different dosages in animal models. At lower doses, it effectively blocks potassium channels without causing significant adverse effects. At higher doses, this compound can exhibit toxic effects, including disruptions in ion homeostasis and cellular toxicity. Threshold effects have been observed, where the compound’s efficacy plateaus at certain concentrations .
Metabolic Pathways
This compound is involved in various metabolic pathways, interacting with enzymes and cofactors that regulate ion transport and cellular metabolism. This compound can affect metabolic flux and metabolite levels by inhibiting or activating specific enzymes. The interactions of this compound with metabolic pathways are crucial for understanding its overall impact on cellular function .
Transport and Distribution
Within cells and tissues, this compound is transported and distributed through specific transporters and binding proteins. These interactions influence its localization and accumulation in different cellular compartments. The transport and distribution of this compound are essential for its effective inhibition of potassium channels and other biochemical activities .
Subcellular Localization
This compound exhibits specific subcellular localization, which can affect its activity and function. Targeting signals and post-translational modifications direct this compound to particular compartments or organelles within the cell. The subcellular localization of this compound is critical for its role in blocking potassium channels and influencing cellular processes .
Preparation Methods
Synthetic Routes and Reaction Conditions: Tetraethylammonium chloride hydrate is produced by the alkylation of triethylamine with ethyl chloride. The reaction typically occurs under controlled conditions to ensure the formation of the desired product .
Industrial Production Methods: In industrial settings, the production of this compound involves large-scale alkylation processes. The reaction is carefully monitored to maintain the purity and yield of the compound. The resulting product is then crystallized and hydrated to obtain the monohydrate or tetrahydrate forms .
Chemical Reactions Analysis
Types of Reactions: Tetraethylammonium chloride hydrate undergoes various chemical reactions, including substitution and complex formation. It is known to block potassium channels non-specifically and inhibit relaxation induced by peroxynitrite .
Common Reagents and Conditions: Common reagents used in reactions with this compound include strong acids and bases, as well as other quaternary ammonium compounds. The reactions are typically carried out under controlled temperature and pressure conditions to ensure the desired outcomes .
Major Products Formed: The major products formed from reactions involving this compound depend on the specific reagents and conditions used. For example, it can form complexes with various metal ions and participate in phase-transfer catalysis .
Scientific Research Applications
Tetraethylammonium chloride hydrate has a wide range of applications in scientific research:
Mechanism of Action
The mechanism of action of tetraethylammonium chloride hydrate involves blocking autonomic ganglia, calcium- and voltage-activated potassium channels, and nicotinic acetylcholine receptors . It can physically enter the pore of potassium channels and block them, thereby inhibiting their function . Additionally, it can either mimic or antagonize the actions of choline and acetylcholine .
Comparison with Similar Compounds
- Tetraethylammonium bromide
- Tetraethylammonium iodide
- Tetramethylammonium chloride
- Tetrabutylammonium chloride
Biological Activity
Tetraethylammonium chloride hydrate (TEAC) is a quaternary ammonium compound with significant biological activity, particularly in pharmacological and physiological research. This article delves into its mechanisms, applications, and relevant case studies, supported by detailed data tables and findings from diverse sources.
TEAC has the molecular formula and appears as a hygroscopic, colorless crystalline solid. It is often encountered in its monohydrate form. The compound is synthesized through the alkylation of triethylamine with ethyl chloride, resulting in tetraethylammonium ions that associate with chloride ions to form the final product .
TEAC acts primarily as a potassium channel blocker . Its mechanism involves competing with acetylcholine at nicotinic receptors, effectively blocking neurotransmission at autonomic ganglia. This property has made it a valuable tool in studying ion channel behavior and neuromuscular transmission .
Key Mechanisms:
- Ganglionic Blocking : TEAC inhibits the action of acetylcholine at nicotinic receptors, leading to reduced neurotransmission.
- Potassium Channel Inhibition : It non-specifically blocks K channels, which has implications for various physiological processes .
Biological Applications
TEAC has been utilized in various biological studies due to its effects on ion channels and neurotransmission. Below are some notable applications:
- Pharmacological Studies : It has been used to explore ganglionic blocking properties and the behavior of K channels in excitable tissues .
- Cardiovascular Research : TEAC has shown potential in improving cardiac and vascular function during early sepsis in animal models .
- Cancer Research : Studies indicate that TEAC induces apoptosis in glioma cells by modulating Bcl-2 family proteins, demonstrating its cytotoxic potential against certain cancer types .
Data Table: Summary of Biological Activities
Biological Activity | Description |
---|---|
Ganglionic Blockade | Competitive antagonist at nicotinic receptors, reducing neurotransmission |
Potassium Channel Blockade | Inhibits K currents, affecting various physiological processes |
Cytotoxic Effects | Induces apoptosis in glioma cells; downregulates Bcl-2, upregulates Bax |
Anti-inflammatory Properties | Reduces inflammatory responses in sepsis models |
Applications in Drug Research | Used as a model compound for studying ion channel behavior |
Case Studies
-
Neuromuscular Transmission Studies :
A study demonstrated that TEAC effectively blocked K currents in rat aorta rings, providing insights into its role as a pharmacological agent for neuromuscular transmission research . -
Cancer Cell Apoptosis :
Research indicated that TEAC could induce apoptosis in glioma cells by altering the expression of key regulatory proteins (Bcl-2 family), suggesting potential therapeutic applications in oncology . -
Sepsis Models :
In animal models of sepsis, TEAC was found to improve hemodynamic properties and reduce inflammation, highlighting its potential utility in critical care settings .
Toxicity and Safety Considerations
While TEAC has valuable biological properties, it is also associated with certain toxic effects. It can cause skin and eye irritation upon contact and should be handled with appropriate personal protective equipment (PPE). The acute toxicity profile is comparable to other quaternary ammonium compounds like tetraethylammonium bromide and iodide .
Properties
IUPAC Name |
tetraethylazanium;chloride;hydrate | |
---|---|---|
Source | PubChem | |
URL | https://pubchem.ncbi.nlm.nih.gov | |
Description | Data deposited in or computed by PubChem | |
InChI |
InChI=1S/C8H20N.ClH.H2O/c1-5-9(6-2,7-3)8-4;;/h5-8H2,1-4H3;1H;1H2/q+1;;/p-1 | |
Source | PubChem | |
URL | https://pubchem.ncbi.nlm.nih.gov | |
Description | Data deposited in or computed by PubChem | |
InChI Key |
CALLTGJPWMIDPC-UHFFFAOYSA-M | |
Source | PubChem | |
URL | https://pubchem.ncbi.nlm.nih.gov | |
Description | Data deposited in or computed by PubChem | |
Canonical SMILES |
CC[N+](CC)(CC)CC.O.[Cl-] | |
Source | PubChem | |
URL | https://pubchem.ncbi.nlm.nih.gov | |
Description | Data deposited in or computed by PubChem | |
Molecular Formula |
C8H22ClNO | |
Source | PubChem | |
URL | https://pubchem.ncbi.nlm.nih.gov | |
Description | Data deposited in or computed by PubChem | |
DSSTOX Substance ID |
DTXSID60583384 | |
Record name | N,N,N-Triethylethanaminium chloride--water (1/1/1) | |
Source | EPA DSSTox | |
URL | https://comptox.epa.gov/dashboard/DTXSID60583384 | |
Description | DSSTox provides a high quality public chemistry resource for supporting improved predictive toxicology. | |
Molecular Weight |
183.72 g/mol | |
Source | PubChem | |
URL | https://pubchem.ncbi.nlm.nih.gov | |
Description | Data deposited in or computed by PubChem | |
CAS No. |
68696-18-4 | |
Record name | N,N,N-Triethylethanaminium chloride--water (1/1/1) | |
Source | EPA DSSTox | |
URL | https://comptox.epa.gov/dashboard/DTXSID60583384 | |
Description | DSSTox provides a high quality public chemistry resource for supporting improved predictive toxicology. | |
Record name | Tetraethylammonium chloride monohydrate | |
Source | European Chemicals Agency (ECHA) | |
URL | https://echa.europa.eu/information-on-chemicals | |
Description | The European Chemicals Agency (ECHA) is an agency of the European Union which is the driving force among regulatory authorities in implementing the EU's groundbreaking chemicals legislation for the benefit of human health and the environment as well as for innovation and competitiveness. | |
Explanation | Use of the information, documents and data from the ECHA website is subject to the terms and conditions of this Legal Notice, and subject to other binding limitations provided for under applicable law, the information, documents and data made available on the ECHA website may be reproduced, distributed and/or used, totally or in part, for non-commercial purposes provided that ECHA is acknowledged as the source: "Source: European Chemicals Agency, http://echa.europa.eu/". Such acknowledgement must be included in each copy of the material. ECHA permits and encourages organisations and individuals to create links to the ECHA website under the following cumulative conditions: Links can only be made to webpages that provide a link to the Legal Notice page. | |
Retrosynthesis Analysis
AI-Powered Synthesis Planning: Our tool employs the Template_relevance Pistachio, Template_relevance Bkms_metabolic, Template_relevance Pistachio_ringbreaker, Template_relevance Reaxys, Template_relevance Reaxys_biocatalysis model, leveraging a vast database of chemical reactions to predict feasible synthetic routes.
One-Step Synthesis Focus: Specifically designed for one-step synthesis, it provides concise and direct routes for your target compounds, streamlining the synthesis process.
Accurate Predictions: Utilizing the extensive PISTACHIO, BKMS_METABOLIC, PISTACHIO_RINGBREAKER, REAXYS, REAXYS_BIOCATALYSIS database, our tool offers high-accuracy predictions, reflecting the latest in chemical research and data.
Strategy Settings
Precursor scoring | Relevance Heuristic |
---|---|
Min. plausibility | 0.01 |
Model | Template_relevance |
Template Set | Pistachio/Bkms_metabolic/Pistachio_ringbreaker/Reaxys/Reaxys_biocatalysis |
Top-N result to add to graph | 6 |
Feasible Synthetic Routes
Q1: What can you tell us about the interactions of tetraethylammonium chloride hydrate with water molecules?
A1: While the provided abstracts don't delve into the specific applications of this compound, one study focuses on its interactions with water molecules. The paper titled "Hydrogen bonding. 11. Infrared study of the water-chloride ion cluster in this compound" [] likely explores the hydrogen bonding interactions between the chloride ion in this compound and water molecules. This suggests the compound's potential use in systems involving aqueous solutions, where understanding these interactions is crucial.
Q2: What are some potential applications of this compound based on its characteristics?
A2: The provided abstracts hint at potential applications based on the compound's properties. For instance, the study on the water-chloride ion cluster [] suggests its relevance in understanding solvation processes and electrolyte behavior in solutions. While not directly addressed in the abstracts, tetraethylammonium salts, including the chloride hydrate, are often used in research as phase-transfer catalysts due to their ability to facilitate reactions between aqueous and organic phases.
Q3: Are there any studies exploring the use of this compound in material science or catalysis?
A3: While the provided abstracts don't directly investigate these applications, one paper, "Selective removal and inactivation of bacteria by nanoparticle composites prepared by surface modification of montmorillonite with quaternary ammonium compounds" [], explores the antibacterial properties of quaternary ammonium compounds. Tetraethylammonium chloride falls under the category of quaternary ammonium compounds. This suggests potential applications in areas like antibacterial surface coatings or water treatment, though further research is needed to confirm its effectiveness in these specific contexts.
Disclaimer and Information on In-Vitro Research Products
Please be aware that all articles and product information presented on BenchChem are intended solely for informational purposes. The products available for purchase on BenchChem are specifically designed for in-vitro studies, which are conducted outside of living organisms. In-vitro studies, derived from the Latin term "in glass," involve experiments performed in controlled laboratory settings using cells or tissues. It is important to note that these products are not categorized as medicines or drugs, and they have not received approval from the FDA for the prevention, treatment, or cure of any medical condition, ailment, or disease. We must emphasize that any form of bodily introduction of these products into humans or animals is strictly prohibited by law. It is essential to adhere to these guidelines to ensure compliance with legal and ethical standards in research and experimentation.