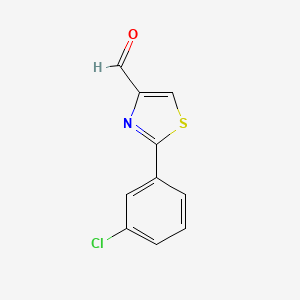
2-(3-Chlorophenyl)thiazole-4-carbaldehyde
Overview
Description
2-(3-Chlorophenyl)thiazole-4-carbaldehyde (CAS: 859850-99-0) is a heterocyclic organic compound featuring a thiazole core substituted with a 3-chlorophenyl group at position 2 and a formyl (-CHO) group at position 3. Its molecular formula is C₁₀H₆ClNOS, with a molecular weight of 223.68 g/mol . The compound is utilized in medicinal chemistry as a building block for drug development, particularly in synthesizing pharmacologically active molecules. It requires storage under inert conditions at 2–8°C due to its aldehyde group's reactivity .
Preparation Methods
Hantzsch Thiazole Synthesis
This classical method involves the condensation of α-haloketones with thioamide derivatives under basic conditions to form the thiazole ring. For 2-(3-Chlorophenyl)thiazole-4-carbaldehyde, the key steps are:
- Starting materials: 3-chlorobenzaldehyde or 3-chlorophenyl derivatives and thioamide.
- Reaction: α-Haloketone (derived from 3-chlorophenyl precursors) reacts with thioamide in the presence of a base (e.g., sodium hydroxide, potassium carbonate).
- Conditions: Heating under reflux in polar solvents to promote cyclization.
- Outcome: Formation of the thiazole ring with the aldehyde group positioned at the 4-position.
This method is widely used due to its straightforwardness and good yields, and it is adaptable for scale-up in industrial settings with continuous flow reactors to optimize reaction control and minimize by-products.
Suzuki Cross-Coupling Followed by Formylation
An alternative modern approach involves the Suzuki coupling reaction between halogenated thiazole intermediates and arylboronic acids:
- Step 1: Preparation of 2-bromo-4-formylthiazole as the halogenated intermediate.
- Step 2: Suzuki coupling with 3-chlorophenylboronic acid in the presence of a palladium catalyst under inert atmosphere (nitrogen).
- Reaction conditions: Use of degassed ethanol and 1,2-dimethoxyethane (1:1), sodium carbonate as base, heating at 100°C for 24 hours.
- Workup: Extraction with ethyl acetate, filtration through Celite, and purification by column chromatography.
Vilsmeier-Haack Formylation
The aldehyde group at the 4-position of the thiazole ring can be introduced or modified via the Vilsmeier-Haack reaction:
- Reactants: Thiazole intermediate without aldehyde group.
- Reagents: N,N-dimethylformamide (DMF) and phosphorus oxychloride (POCl3).
- Conditions: Controlled temperature, typically 0–5°C initially, then warming to room temperature or slight heating.
- Mechanism: Formation of the Vilsmeier reagent from DMF and POCl3, which electrophilically attacks the thiazole ring to introduce the formyl group.
- Outcome: High regioselectivity for the 4-position aldehyde substitution.
This method is especially valuable for late-stage functionalization and is compatible with various substituents on the thiazole ring.
Method | Key Reactants | Reaction Conditions | Advantages | Limitations |
---|---|---|---|---|
Hantzsch Thiazole Synthesis | α-Haloketone, Thioamide | Base (NaOH/K2CO3), reflux, polar solvent | Simple, scalable, good yields | May require purification steps |
Suzuki Cross-Coupling + Formylation | 2-Bromo-4-formylthiazole, 3-chlorophenylboronic acid, Pd catalyst | EtOH/DME solvent, Na2CO3, 100°C, 24 h | High purity, selective substitution | Requires palladium catalyst, longer reaction time |
Vilsmeier-Haack Formylation | Thiazole intermediate, DMF, POCl3 | 0–5°C to RT, controlled addition | Regioselective, effective for aldehyde introduction | Sensitive reagents, moisture sensitive |
- Studies have shown that the Hantzsch synthesis route benefits from the use of bases such as potassium carbonate to improve yield and reduce side reactions.
- The Suzuki coupling method, while more complex, allows for the introduction of diverse aryl groups, enabling structural analog development for biological activity screening.
- The Vilsmeier-Haack reaction conditions must be carefully optimized to avoid over-formylation or degradation of sensitive thiazole rings.
- Industrial scale synthesis often employs continuous flow reactors for the Hantzsch method to enhance reproducibility and safety.
The preparation of this compound is well-established through several synthetic routes, each with unique advantages:
- The Hantzsch thiazole synthesis offers a classical, efficient route suitable for scale-up.
- Suzuki coupling provides structural versatility and high purity for advanced applications.
- Vilsmeier-Haack formylation is a powerful tool for aldehyde group introduction with regioselectivity.
These methods are supported by detailed reaction condition optimizations and have been validated in both laboratory and industrial contexts, ensuring reliable access to this valuable compound for further biochemical and pharmaceutical research.
Chemical Reactions Analysis
Types of Reactions: 2-(3-Chlorophenyl)thiazole-4-carbaldehyde undergoes various chemical reactions, including:
Oxidation: The aldehyde group can be oxidized to form the corresponding carboxylic acid.
Reduction: The aldehyde group can be reduced to form the corresponding alcohol.
Substitution: The thiazole ring can undergo electrophilic substitution reactions, particularly at the 5-position.
Common Reagents and Conditions:
Oxidation: Common oxidizing agents include potassium permanganate (KMnO4) and chromium trioxide (CrO3).
Reduction: Reducing agents such as sodium borohydride (NaBH4) or lithium aluminum hydride (LiAlH4) are typically used.
Major Products Formed:
Oxidation: 2-(3-Chlorophenyl)thiazole-4-carboxylic acid.
Reduction: 2-(3-Chlorophenyl)thiazole-4-methanol.
Substitution: Various substituted thiazole derivatives depending on the electrophile used.
Scientific Research Applications
2-(3-Chlorophenyl)thiazole-4-carbaldehyde is a chemical compound with diverse applications in scientific research, including pharmaceutical development, agricultural chemistry, material science, biological research, and analytical chemistry . It is used as an intermediate in synthesizing more complex thiazole derivatives and heterocyclic compounds. The chlorophenyl group contributes to enhanced color properties, making it useful in the production of dyes and pigments .
Applications
Pharmaceutical Development: this compound is a key intermediate in synthesizing pharmaceuticals, particularly those targeting specific diseases, thereby enhancing the efficiency of drug discovery processes .
Agricultural Chemistry: It is utilized in formulating agrochemicals, providing solutions for pest control and crop protection, which is crucial for sustainable agriculture .
Material Science: The compound is explored for its potential in developing novel materials like polymers and coatings that require specific chemical properties for enhanced performance .
Biological Research: this compound plays a role in biological studies, especially in understanding enzyme interactions and cellular processes, assisting researchers in discovering new therapeutic targets . Studies show that the compound can influence cell function by affecting cell signaling pathways, gene expression, and cellular metabolism. For instance, it has been found to induce apoptosis in cancer cells by activating the intrinsic apoptotic pathway.
Analytical Chemistry: This chemical is utilized in analytical methods, including chromatography, to identify and quantify other substances, ensuring quality control in manufacturing processes .
Scientific Research
- Chemistry: this compound is used as an intermediate in synthesizing more complex thiazole derivatives and heterocyclic compounds.
- Biology: The compound is studied for its potential biological activities, including antimicrobial and anticancer properties.
- Medicine: Research is ongoing to explore its potential as a pharmacophore in drug design, particularly for targeting specific enzymes and receptors.
- Industry: It is used in developing new materials with specific electronic and optical properties. Its application extends to the production of dyes and pigments, where its chlorophenyl group contributes to enhanced color properties .
Biochemical Analysis
This compound plays a significant role in biochemical reactions, particularly in the context of its interactions with enzymes, proteins, and other biomolecules. This compound has been shown to interact with various enzymes, including topoisomerase IV, which is involved in DNA replication and repair.
Products Formed
- Oxidation: 2-(3-Chlorophenyl)thiazole-4-carboxylic acid.
- Reduction: 2-(3-Chlorophenyl)thiazole-4-methanol.
- Substitution: Various substituted thiazole derivatives, depending on the electrophile used.
Mechanism of Action
The mechanism of action of 2-(3-Chlorophenyl)thiazole-4-carbaldehyde involves its interaction with specific molecular targets. For instance, it can act as an inhibitor of certain enzymes by binding to their active sites, thereby blocking their activity. The thiazole ring and the aldehyde group play crucial roles in its binding affinity and specificity . Additionally, the compound may interact with cellular pathways involved in oxidative stress and apoptosis, contributing to its potential therapeutic effects .
Comparison with Similar Compounds
Comparison with Structural Analogs
Halogen-Substituted Thiazole Derivatives
The following table compares 2-(3-Chlorophenyl)thiazole-4-carbaldehyde with closely related halogenated analogs:
Key Observations :
- Para-substituted analogs (e.g., 4-chloro) may exhibit altered electronic distributions, affecting crystallinity and solubility .
- Molecular Weight : Bromine substitution (as in SC-22242) increases molecular weight by ~44 g/mol compared to chlorine, which could enhance lipophilicity and impact pharmacokinetic properties .
- Hazard Profile : The target compound’s hazards (H302, H317, H319) highlight risks of oral toxicity, skin sensitization, and eye irritation, but analogous hazards for other derivatives remain unreported .
Benzothiazole and Pyrazole Derivatives
N-(6-Trifluoromethylbenzothiazole-2-yl)-2-(3-chlorophenyl)acetamide
- Core Structure : Benzothiazole (fused benzene-thiazole ring) vs. simple thiazole.
- Substituents : Trifluoromethyl (-CF₃) at position 6 and 3-chlorophenylacetamide at position 2.
- Comparison: The benzothiazole core enhances aromaticity and stability compared to thiazole.
5-(3-Chlorophenylsulfanyl)-1-methyl-3-trifluoromethyl-1H-pyrazole-4-carbaldehyde
- Core Structure : Pyrazole ring with a sulfanyl (-S-) linkage to 3-chlorophenyl.
- Substituents : Trifluoromethyl (-CF₃) at position 3 and carbaldehyde at position 4.
- The trifluoromethyl group enhances metabolic stability compared to chlorine .
Physicochemical and Reactivity Comparisons
- Solubility : The meta-chloro substituent may reduce aqueous solubility compared to para-substituted analogs due to asymmetric crystal packing. Brominated derivatives likely exhibit lower solubility owing to increased molecular weight .
- Reactivity : The aldehyde group in all analogs serves as an electrophilic site for condensation reactions. However, electron-withdrawing groups (e.g., -CF₃ in benzothiazole derivatives) may accelerate reaction rates with nucleophiles .
Structural Analysis and Crystallography
Crystallographic studies using programs like SHELXL (for refinement) and ORTEP-3 (for visualization) could elucidate differences in bond lengths and angles between the target compound and its analogs. For example, meta-substituted phenyl groups may introduce torsional strain compared to para-substituted derivatives, influencing molecular packing .
Biological Activity
2-(3-Chlorophenyl)thiazole-4-carbaldehyde is a compound of significant interest in medicinal chemistry due to its diverse biological activities. This article explores its antibacterial, anticancer, and anti-inflammatory properties, supported by relevant research findings and case studies.
Chemical Structure and Properties
This compound features a thiazole ring, which is known for its ability to interact with biological targets due to its electron-withdrawing properties. The presence of the 3-chlorophenyl group enhances its lipophilicity and biological activity.
Antibacterial Activity
Research has demonstrated that thiazole derivatives, including this compound, exhibit significant antibacterial properties.
- Minimum Inhibitory Concentration (MIC) : Studies indicate that compounds within this class show MIC values ranging from 40 to 50 µg/mL against various bacterial strains, including E. faecalis, P. aeruginosa, S. typhi, and K. pneumoniae .
- Comparison with Standards : The antibacterial activity is comparable to standard antibiotics like ceftriaxone, with inhibition zone diameters reaching up to 30 mm against certain pathogens .
Anticancer Activity
The anticancer potential of this compound has been evaluated through various in vitro studies.
- Cell Lines Tested : Its cytotoxic effects have been assessed against human cancer cell lines such as MCF-7 (breast cancer) and HepG2 (liver cancer).
- Cytotoxicity Results : The compound demonstrated notable cytotoxicity with an IC50 value of approximately 5.36 µg/mL against MCF-7 cells, indicating a strong antiproliferative effect . The mechanism of action involves inducing apoptosis, as evidenced by increased levels of pro-apoptotic markers like Bax and caspase-9 in treated cells .
Cell Line | IC50 (µg/mL) | Mechanism of Action |
---|---|---|
MCF-7 | 5.36 | Induces apoptosis |
HepG2 | 10.10 | Cell cycle arrest at G2/M phase |
Anti-inflammatory Activity
In addition to its antibacterial and anticancer properties, this compound has shown potential anti-inflammatory effects.
- Mechanism : The compound may inhibit pro-inflammatory cytokines and pathways, contributing to its therapeutic profile in conditions characterized by inflammation .
Case Studies
- Cytotoxicity Study : A study evaluated the effects of various thiazole derivatives on MCF-7 cells, revealing that modifications in the structure significantly influenced their cytotoxic potency. The introduction of different substituents on the thiazole ring enhanced activity against cancer cells .
- Antibacterial Efficacy : Another investigation focused on the antibacterial efficacy of thiazole derivatives against clinical isolates of resistant bacteria, demonstrating that compounds like this compound could serve as promising leads for new antibiotics .
Q & A
Basic Research Questions
Q. What synthetic methodologies are most effective for preparing 2-(3-chlorophenyl)thiazole-4-carbaldehyde, and how can reaction conditions be optimized?
The compound can be synthesized via Hantzsch condensation, where thiosemicarbazones react with halogenated ketones (e.g., 2-bromo-4’-chloroacetophenone) under reflux conditions . Catalyst-free approaches, such as Knöevenagel condensation in PEG-400, are also viable for minimizing side reactions and improving yields . Optimization should focus on solvent selection (e.g., glacial acetic acid vs. PEG-400), temperature control, and reaction monitoring via TLC or HPLC to isolate intermediates.
Q. How can structural elucidation of this compound be performed with high accuracy?
Single-crystal X-ray diffraction (SC-XRD) using SHELX programs (e.g., SHELXL for refinement) is the gold standard for resolving bond angles, stereochemistry, and intermolecular interactions . Complement this with computational methods (DFT or semi-empirical MO calculations) to validate electronic properties and compare experimental vs. theoretical data . NMR (¹H/¹³C) and HRMS should confirm functional groups and molecular weight, respectively .
Q. What analytical techniques are recommended for assessing the purity of this compound in research settings?
Use HPLC with a C18 column and UV detection (λ = 254 nm) to quantify impurities. Pair this with LC-MS to confirm molecular ion peaks and detect degradation products . For trace metal analysis, ICP-MS is advised if transition-metal catalysts (e.g., Pd/C) are used in synthesis.
Advanced Research Questions
Q. How can researchers resolve contradictions in crystallographic data for halogenated thiazole derivatives?
Discrepancies in bond lengths or packing motifs may arise from polymorphism or twinning. Employ twin refinement in SHELXL and compare results with computational models (e.g., Mercury CSD) . Validate using complementary techniques like PXRD to assess bulk crystallinity and DSC to detect phase transitions .
Q. What strategies are effective for evaluating the biological activity of this compound in drug discovery?
Design in vitro assays targeting specific enzymes or receptors (e.g., kinase inhibition). Use molecular docking (AutoDock Vina, Schrödinger) to predict binding modes to active sites, followed by MD simulations to assess stability . For in vitro validation, employ dose-response curves (IC₅₀ determination) and selectivity profiling against related targets to minimize off-target effects .
Q. How can computational models improve the synthesis and functionalization of this compound?
DFT calculations (Gaussian, ORCA) can predict regioselectivity in electrophilic substitution reactions at the thiazole ring. For example, calculate Fukui indices to identify reactive sites for aldehyde group modifications . QSAR models can also guide derivatization by linking structural features (e.g., chloro-substitution patterns) to bioactivity .
Q. What mechanistic insights explain the reactivity of the aldehyde group in cross-coupling reactions?
The aldehyde’s electrophilicity facilitates nucleophilic additions (e.g., Grignard reagents) or condensations (e.g., with hydrazines to form hydrazones). Study kinetic isotope effects (KIE) or isotopic labeling (¹³C-aldehyde) to track reaction pathways . In situ IR spectroscopy can monitor intermediate formation during catalytic cycles.
Q. Methodological Considerations
Q. How should researchers address challenges in reproducing synthetic protocols for this compound?
Document exact solvent grades (e.g., anhydrous vs. technical grade), purification methods (column chromatography vs. recrystallization), and moisture-sensitive steps. Cross-validate yields and purity data with independent labs . For air-sensitive reactions, use Schlenk-line techniques or gloveboxes.
Q. What approaches are recommended for analyzing intermolecular interactions in crystalline forms?
Hirshfeld surface analysis (CrystalExplorer) quantifies interactions like C–H···O, π-π stacking, or halogen bonds. Compare interaction fingerprints with analogs (e.g., 2-(4-chlorophenyl)thiazole derivatives) to identify packing trends influenced by substituent position .
Q. How can SAR studies be structured to explore the impact of the 3-chlorophenyl group on bioactivity?
Synthesize analogs with substituents varying in electronegativity (e.g., 3-fluoro, 3-bromo) or steric bulk. Test against a panel of biological targets (e.g., antimicrobial, anticancer assays) and correlate activity with Hammett constants or steric parameters . Use CoMFA or CoMSIA models to visualize 3D pharmacophore requirements.
Properties
IUPAC Name |
2-(3-chlorophenyl)-1,3-thiazole-4-carbaldehyde | |
---|---|---|
Source | PubChem | |
URL | https://pubchem.ncbi.nlm.nih.gov | |
Description | Data deposited in or computed by PubChem | |
InChI |
InChI=1S/C10H6ClNOS/c11-8-3-1-2-7(4-8)10-12-9(5-13)6-14-10/h1-6H | |
Source | PubChem | |
URL | https://pubchem.ncbi.nlm.nih.gov | |
Description | Data deposited in or computed by PubChem | |
InChI Key |
XMXWYXGIKFBCGR-UHFFFAOYSA-N | |
Source | PubChem | |
URL | https://pubchem.ncbi.nlm.nih.gov | |
Description | Data deposited in or computed by PubChem | |
Canonical SMILES |
C1=CC(=CC(=C1)Cl)C2=NC(=CS2)C=O | |
Source | PubChem | |
URL | https://pubchem.ncbi.nlm.nih.gov | |
Description | Data deposited in or computed by PubChem | |
Molecular Formula |
C10H6ClNOS | |
Source | PubChem | |
URL | https://pubchem.ncbi.nlm.nih.gov | |
Description | Data deposited in or computed by PubChem | |
DSSTOX Substance ID |
DTXSID80594553 | |
Record name | 2-(3-Chlorophenyl)-1,3-thiazole-4-carbaldehyde | |
Source | EPA DSSTox | |
URL | https://comptox.epa.gov/dashboard/DTXSID80594553 | |
Description | DSSTox provides a high quality public chemistry resource for supporting improved predictive toxicology. | |
Molecular Weight |
223.68 g/mol | |
Source | PubChem | |
URL | https://pubchem.ncbi.nlm.nih.gov | |
Description | Data deposited in or computed by PubChem | |
CAS No. |
859850-99-0 | |
Record name | 2-(3-Chlorophenyl)-4-thiazolecarboxaldehyde | |
Source | CAS Common Chemistry | |
URL | https://commonchemistry.cas.org/detail?cas_rn=859850-99-0 | |
Description | CAS Common Chemistry is an open community resource for accessing chemical information. Nearly 500,000 chemical substances from CAS REGISTRY cover areas of community interest, including common and frequently regulated chemicals, and those relevant to high school and undergraduate chemistry classes. This chemical information, curated by our expert scientists, is provided in alignment with our mission as a division of the American Chemical Society. | |
Explanation | The data from CAS Common Chemistry is provided under a CC-BY-NC 4.0 license, unless otherwise stated. | |
Record name | 2-(3-Chlorophenyl)-1,3-thiazole-4-carbaldehyde | |
Source | EPA DSSTox | |
URL | https://comptox.epa.gov/dashboard/DTXSID80594553 | |
Description | DSSTox provides a high quality public chemistry resource for supporting improved predictive toxicology. | |
Disclaimer and Information on In-Vitro Research Products
Please be aware that all articles and product information presented on BenchChem are intended solely for informational purposes. The products available for purchase on BenchChem are specifically designed for in-vitro studies, which are conducted outside of living organisms. In-vitro studies, derived from the Latin term "in glass," involve experiments performed in controlled laboratory settings using cells or tissues. It is important to note that these products are not categorized as medicines or drugs, and they have not received approval from the FDA for the prevention, treatment, or cure of any medical condition, ailment, or disease. We must emphasize that any form of bodily introduction of these products into humans or animals is strictly prohibited by law. It is essential to adhere to these guidelines to ensure compliance with legal and ethical standards in research and experimentation.