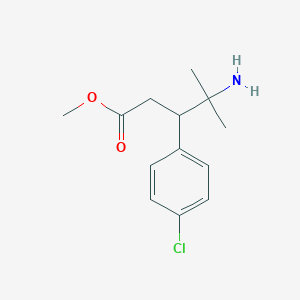
Methyl 4-amino-3-(4-chlorophenyl)-4-methylpentanoate
- Click on QUICK INQUIRY to receive a quote from our team of experts.
- With the quality product at a COMPETITIVE price, you can focus more on your research.
Overview
Description
Methyl 4-amino-3-(4-chlorophenyl)-4-methylpentanoate is an organic compound that belongs to the class of amino acid derivatives. This compound is characterized by the presence of an amino group, a chlorophenyl group, and a methyl ester group. It is used in various scientific research applications due to its unique chemical properties.
Preparation Methods
Synthetic Routes and Reaction Conditions
The synthesis of Methyl 4-amino-3-(4-chlorophenyl)-4-methylpentanoate typically involves the reaction of 4-chlorobenzaldehyde with methyl acetoacetate in the presence of ammonium acetate. The reaction is carried out under reflux conditions, and the product is purified by recrystallization.
Industrial Production Methods
In industrial settings, the production of this compound involves large-scale reactions using similar reagents and conditions. The process is optimized for higher yields and purity, often involving advanced purification techniques such as column chromatography and crystallization.
Chemical Reactions Analysis
Types of Reactions
Methyl 4-amino-3-(4-chlorophenyl)-4-methylpentanoate undergoes various chemical reactions, including:
Oxidation: The compound can be oxidized to form corresponding ketones or carboxylic acids.
Reduction: Reduction reactions can convert the compound into its corresponding alcohols or amines.
Substitution: The chlorophenyl group can undergo nucleophilic substitution reactions, leading to the formation of various derivatives.
Common Reagents and Conditions
Oxidation: Common oxidizing agents include potassium permanganate and chromium trioxide.
Reduction: Reducing agents such as lithium aluminum hydride and sodium borohydride are commonly used.
Substitution: Nucleophiles such as amines, thiols, and alcohols can be used in substitution reactions.
Major Products Formed
Oxidation: Formation of ketones or carboxylic acids.
Reduction: Formation of alcohols or amines.
Substitution: Formation of various substituted derivatives.
Scientific Research Applications
Methyl 4-amino-3-(4-chlorophenyl)-4-methylpentanoate is used in various scientific research applications, including:
Chemistry: As a building block for the synthesis of more complex molecules.
Biology: In the study of enzyme-substrate interactions and protein-ligand binding.
Medicine: Potential use in the development of pharmaceutical compounds.
Industry: Used in the synthesis of specialty chemicals and materials.
Mechanism of Action
The mechanism of action of Methyl 4-amino-3-(4-chlorophenyl)-4-methylpentanoate involves its interaction with specific molecular targets. The compound can bind to enzymes and receptors, modulating their activity. The pathways involved include inhibition or activation of enzymatic reactions, leading to various biological effects.
Comparison with Similar Compounds
Similar Compounds
- Methyl 4-amino-3-(4-bromophenyl)-4-methylpentanoate
- Methyl 4-amino-3-(4-fluorophenyl)-4-methylpentanoate
- Methyl 4-amino-3-(4-methylphenyl)-4-methylpentanoate
Uniqueness
Methyl 4-amino-3-(4-chlorophenyl)-4-methylpentanoate is unique due to the presence of the chlorophenyl group, which imparts distinct chemical and biological properties. This makes it a valuable compound for various research applications, distinguishing it from its analogs with different substituents.
Properties
Molecular Formula |
C13H18ClNO2 |
---|---|
Molecular Weight |
255.74 g/mol |
IUPAC Name |
methyl 4-amino-3-(4-chlorophenyl)-4-methylpentanoate |
InChI |
InChI=1S/C13H18ClNO2/c1-13(2,15)11(8-12(16)17-3)9-4-6-10(14)7-5-9/h4-7,11H,8,15H2,1-3H3 |
InChI Key |
JZWKNFMLYHWTOQ-UHFFFAOYSA-N |
Canonical SMILES |
CC(C)(C(CC(=O)OC)C1=CC=C(C=C1)Cl)N |
Origin of Product |
United States |
Disclaimer and Information on In-Vitro Research Products
Please be aware that all articles and product information presented on BenchChem are intended solely for informational purposes. The products available for purchase on BenchChem are specifically designed for in-vitro studies, which are conducted outside of living organisms. In-vitro studies, derived from the Latin term "in glass," involve experiments performed in controlled laboratory settings using cells or tissues. It is important to note that these products are not categorized as medicines or drugs, and they have not received approval from the FDA for the prevention, treatment, or cure of any medical condition, ailment, or disease. We must emphasize that any form of bodily introduction of these products into humans or animals is strictly prohibited by law. It is essential to adhere to these guidelines to ensure compliance with legal and ethical standards in research and experimentation.