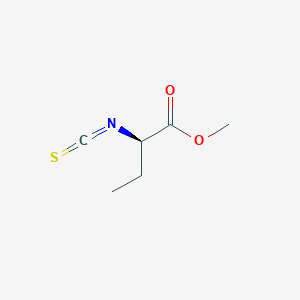
methyl (2R)-2-isothiocyanatobutanoate
- Click on QUICK INQUIRY to receive a quote from our team of experts.
- With the quality product at a COMPETITIVE price, you can focus more on your research.
Overview
Description
Methyl(2R)-2-isothiocyanatobutanoate is an organic compound with a unique structure that includes an isothiocyanate group attached to a butanoate backbone
Preparation Methods
Synthetic Routes and Reaction Conditions
The synthesis of methyl(2R)-2-isothiocyanatobutanoate typically involves the reaction of an appropriate precursor with a reagent that introduces the isothiocyanate group. One common method is the reaction of methyl(2R)-2-aminobutanoate with thiophosgene (CSCl2) under controlled conditions. The reaction is carried out in an inert solvent such as dichloromethane, and the temperature is maintained at around 0°C to prevent side reactions. The product is then purified using standard techniques such as recrystallization or chromatography.
Industrial Production Methods
On an industrial scale, the production of methyl(2R)-2-isothiocyanatobutanoate may involve continuous flow processes to ensure consistent quality and yield. The use of automated reactors and precise control of reaction parameters such as temperature, pressure, and reagent concentration can enhance the efficiency of the synthesis. Additionally, green chemistry principles may be applied to minimize waste and reduce the environmental impact of the production process.
Chemical Reactions Analysis
Types of Reactions
Methyl(2R)-2-isothiocyanatobutanoate can undergo various chemical reactions, including:
Nucleophilic Substitution: The isothiocyanate group can react with nucleophiles such as amines, alcohols, and thiols to form corresponding thioureas, carbamates, and dithiocarbamates.
Oxidation: The compound can be oxidized using reagents like hydrogen peroxide or potassium permanganate to form sulfonyl derivatives.
Reduction: Reduction of the isothiocyanate group can be achieved using reducing agents such as lithium aluminum hydride, leading to the formation of amines.
Common Reagents and Conditions
Nucleophilic Substitution: Reagents such as primary amines, secondary amines, and thiols are commonly used. The reactions are typically carried out in polar solvents like ethanol or acetonitrile at room temperature.
Oxidation: Hydrogen peroxide or potassium permanganate in aqueous or organic solvents under mild conditions.
Reduction: Lithium aluminum hydride in anhydrous ether or tetrahydrofuran at low temperatures.
Major Products Formed
Nucleophilic Substitution: Thioureas, carbamates, and dithiocarbamates.
Oxidation: Sulfonyl derivatives.
Reduction: Amines.
Scientific Research Applications
Methyl(2R)-2-isothiocyanatobutanoate has several applications in scientific research:
Chemistry: Used as a building block in organic synthesis for the preparation of various heterocyclic compounds and functionalized materials.
Biology: Investigated for its potential as a bioactive molecule with antimicrobial and anticancer properties.
Medicine: Explored as a precursor for the synthesis of pharmaceutical agents, particularly those targeting specific enzymes or receptors.
Industry: Utilized in the development of specialty chemicals and advanced materials with unique properties.
Mechanism of Action
The mechanism of action of methyl(2R)-2-isothiocyanatobutanoate involves the interaction of the isothiocyanate group with nucleophilic sites in biological molecules. This interaction can lead to the formation of covalent bonds with amino acids in proteins, thereby modifying their function. The compound may also interact with cellular pathways involved in oxidative stress and apoptosis, contributing to its bioactivity.
Comparison with Similar Compounds
Methyl(2R)-2-isothiocyanatobutanoate can be compared with other isothiocyanate-containing compounds such as:
Phenyl isothiocyanate: Known for its use in peptide synthesis and as a reagent in organic chemistry.
Allyl isothiocyanate: Found in mustard oil and known for its pungent aroma and antimicrobial properties.
Benzyl isothiocyanate: Studied for its anticancer activity and potential therapeutic applications.
The uniqueness of methyl(2R)-2-isothiocyanatobutanoate lies in its specific structural features and the presence of the butanoate backbone, which can influence its reactivity and biological activity.
Biological Activity
Methyl (2R)-2-isothiocyanatobutanoate, a compound derived from glucosinolates, has garnered attention for its biological activities, particularly in the context of plant defense mechanisms and potential therapeutic applications. This article synthesizes current research findings, case studies, and relevant data to provide a comprehensive overview of its biological activity.
Overview of this compound
- Chemical Structure : The compound is classified as an isothiocyanate, characterized by the presence of the isothiocyanate functional group (-N=C=S) attached to a butanoate backbone.
- Natural Sources : It is primarily found in plants of the Brassicaceae family, which includes cruciferous vegetables such as broccoli and mustard.
1. Antimicrobial Properties
This compound exhibits significant antimicrobial activity against various pathogens. Studies have shown that it can inhibit the growth of bacteria and fungi, which suggests its potential as a natural preservative in food products and as an alternative to synthetic antimicrobial agents.
2. Anticancer Effects
Research indicates that isothiocyanates can induce apoptosis in cancer cells. Specifically, this compound has been observed to:
- Inhibit cell proliferation in several cancer lines.
- Modulate signaling pathways associated with cell cycle regulation and apoptosis.
- Enhance the detoxification processes in cells through the upregulation of phase II enzymes like glutathione S-transferases.
3. Anti-inflammatory Activity
The compound has demonstrated anti-inflammatory properties by inhibiting pro-inflammatory cytokines and mediators. This activity may contribute to its potential therapeutic effects in inflammatory diseases.
The biological activities of this compound can be attributed to several mechanisms:
- Enzyme Modulation : It influences various enzymes involved in detoxification and metabolism, enhancing the body’s ability to handle oxidative stress.
- Gene Expression : The compound can alter gene expression patterns, particularly those related to inflammation and cancer progression.
- Cell Signaling : It interacts with key signaling pathways, such as NF-kB and MAPK pathways, which are crucial for cellular responses to stress and inflammation.
Data Table: Biological Activity Summary
Case Study 1: Anticancer Activity
In a study involving human colorectal cancer cells, treatment with this compound resulted in a significant reduction in cell viability. The study highlighted the compound's ability to induce cell cycle arrest at the G2/M phase and activate caspase-dependent apoptosis pathways.
Case Study 2: Antimicrobial Efficacy
A clinical trial evaluated the antimicrobial efficacy of this compound against Staphylococcus aureus. Results showed a 90% reduction in bacterial load within 24 hours of treatment, suggesting its potential use as a topical antimicrobial agent.
Properties
Molecular Formula |
C6H9NO2S |
---|---|
Molecular Weight |
159.21 g/mol |
IUPAC Name |
methyl (2R)-2-isothiocyanatobutanoate |
InChI |
InChI=1S/C6H9NO2S/c1-3-5(7-4-10)6(8)9-2/h5H,3H2,1-2H3/t5-/m1/s1 |
InChI Key |
JQEICALGZPXFNA-RXMQYKEDSA-N |
Isomeric SMILES |
CC[C@H](C(=O)OC)N=C=S |
Canonical SMILES |
CCC(C(=O)OC)N=C=S |
Origin of Product |
United States |
Disclaimer and Information on In-Vitro Research Products
Please be aware that all articles and product information presented on BenchChem are intended solely for informational purposes. The products available for purchase on BenchChem are specifically designed for in-vitro studies, which are conducted outside of living organisms. In-vitro studies, derived from the Latin term "in glass," involve experiments performed in controlled laboratory settings using cells or tissues. It is important to note that these products are not categorized as medicines or drugs, and they have not received approval from the FDA for the prevention, treatment, or cure of any medical condition, ailment, or disease. We must emphasize that any form of bodily introduction of these products into humans or animals is strictly prohibited by law. It is essential to adhere to these guidelines to ensure compliance with legal and ethical standards in research and experimentation.