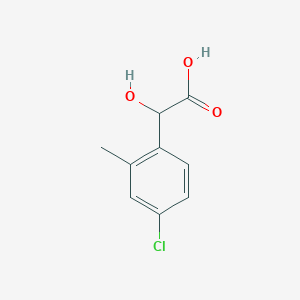
4-Chloro-2-methylmandelic acid
- Click on QUICK INQUIRY to receive a quote from our team of experts.
- With the quality product at a COMPETITIVE price, you can focus more on your research.
Overview
Description
4-Chloro-2-methylmandelic acid (hypothetical structure based on nomenclature rules) is a substituted mandelic acid derivative. Mandelic acid (α-hydroxybenzeneacetic acid) is a well-known aromatic α-hydroxy acid with a phenyl group and a hydroxyl group on the adjacent carbon. In this compound, the phenyl ring is substituted with a chlorine atom at the 4-position and a methyl group at the 2-position (ortho to the hydroxyl-bearing carbon).
Preparation Methods
Preparation Methods of 4-Chloro-2-methylmandelic Acid
General Synthetic Routes for Substituted Mandelic Acids
Substituted mandelic acids such as 4-chloromandelic acid and 2-methylmandelic acid are commonly synthesized from their corresponding substituted benzaldehydes through cyanohydrin intermediates or via dichlorocarbene insertion methods.
- Cyanohydrin Route : This involves conversion of substituted benzaldehydes to cyanohydrins, followed by hydrolysis to yield the mandelic acid derivative.
- Dichlorocarbene Route : This method uses chloroform and strong base (e.g., sodium hydroxide) in the presence of phase transfer catalysts to generate dichlorocarbene, which reacts with substituted benzaldehydes to form mandelic acids.
These methods have been applied to various substituted mandelic acids including chloro- and methyl-substituted derivatives.
Specific Preparation of this compound
Given the substitution pattern, the synthesis can be approached by starting with 4-chloro-2-methylbenzaldehyde as the key precursor. The preparation typically follows:
Step 1: Formation of Cyanohydrin Intermediate
- React 4-chloro-2-methylbenzaldehyde with sodium cyanide and acid catalyst under controlled temperature to form the cyanohydrin.
Step 2: Hydrolysis of Cyanohydrin
- The cyanohydrin is hydrolyzed under acidic or basic conditions to yield this compound.
Alternatively, the dichlorocarbene method can be used:
- React 4-chloro-2-methylbenzaldehyde with chloroform and sodium hydroxide in the presence of a phase transfer catalyst such as benzyltriethylammonium chloride.
- Maintain temperature below 60°C to avoid side reactions.
- After reaction completion, acidify the mixture to precipitate the mandelic acid derivative.
- Extract and purify by recrystallization.
Reaction Conditions and Optimization
From studies on similar substituted mandelic acids, the following parameters are critical:
Purification and Characterization
- The crude product is typically purified by recrystallization from dichloromethane or ethyl acetate.
- Polymorphism is common in substituted mandelic acids, affecting crystallization and purity.
- Characterization includes melting point determination, NMR spectroscopy, and elemental analysis.
Summary Table: Preparation Methods for this compound and Related Compounds
Research Notes and Considerations
- The dichlorocarbene route is widely used for substituted mandelic acids but yields can be inconsistent due to sensitivity to reaction conditions and substituent effects.
- Polymorphism in mandelic acid derivatives can influence crystallization and purity, thus impacting reproducibility.
- Chlorination methods using sulfur oxychloride provide efficient pathways to acid chlorides, which can be intermediates for further functionalization of mandelic acid derivatives.
- Safety considerations are critical when handling reagents like sulfur oxychloride and dichlorocarbene precursors due to toxicity and volatility.
Chemical Reactions Analysis
Oxidation Mechanism and Kinetics
Transition State Analysis
The oxidation proceeds via a carbocationic transition state, supported by solvent effects and linear free energy relationships (r² = 0.9188) . The hydride-ion transfer mechanism involves chromate ester intermediates, with the α-C–H bond cleavage as the rate-determining step.
Kinetic Isotope Effects
Protio- and deuteriomandelic acid studies show temperature-dependent isotope effects, confirming a symmetrical transition state.
Esterification and Derivatization
Alcohol Esterification
4-Chloro-2-methylmandelic acid undergoes esterification with alcohols (e.g., n-butanol) using salicylaldehyde as a catalyst. The reaction is monitored by TLC and NMR, yielding products like n-butyl mandelate .
Analytical Data
Ester derivatives exhibit characteristic NMR shifts (δ 5.24 ppm for CH₂OH) and mass spectral fragments (m/z 242 for methyl ester) .
Enzymatic Interactions and Biological Activity
Enzyme Inhibition
The compound inhibits enzymes involved in plant growth regulation, functioning as a synthetic auxin analog. This activity is attributed to the electron-withdrawing chlorine substituent, which enhances binding affinity to target enzymes.
Growth Regulation
As a plant growth regulator, it promotes cell elongation and division while suppressing weed growth without significantly affecting crops.
Electrophilic Aromatic Substitution
Reactivity Trends
The chloro substituent directs electrophilic substitution to the ortho and para positions due to its electron-withdrawing nature. Substitution patterns are influenced by steric effects from the methyl group at position 2.
Mechanistic Insights
The transition state involves partial positive charge stabilization at the reactive positions, consistent with the carbocation-like intermediates observed in oxidation reactions .
Solvent Effects on Reaction Rates
Linear Solvation Energy Relationships
Oxidation rates correlate with solvent polarity (p*) and hydrogen bond acceptor basicity (β), as described by Kamlet’s equation:
logk2=A0+p⋅π∗+b⋅β
This highlights the role of solvent in stabilizing transition states .
Structural and Spectroscopic Characteristics
NMR and Mass Spectrometry
Key NMR signals include δ 7.43–7.30 ppm (aromatic protons) and δ 5.24 ppm (CH₂OH). Mass spectrometry shows prominent fragments at m/z 242 (M⁺) and m/z 107 (base peak) .
Chirality and Separation
Chiral resolution studies demonstrate enantiomer elution reversal on permethylated cyclodextrins, with selectivity influenced by substituent position and solvent interactions .
Analytical Determination in Environmental Samples
LC-MS Methods
Sensitive detection limits (e.g., 40 ng/L for MCPA analogs) are achieved via electrospray tandem mass spectrometry, enabling quantitation in water and soil after solid-phase extraction .
Scientific Research Applications
2-(4-chloro-2-methylphenyl)-2-hydroxyacetic acid has several scientific research applications:
Chemistry: It is used as an intermediate in the synthesis of various organic compounds.
Biology: The compound is studied for its potential biological activity and interactions with biomolecules.
Medicine: Research is ongoing to explore its potential therapeutic effects and applications in drug development.
Industry: It is used in the production of herbicides and other agrochemicals.
Mechanism of Action
The mechanism of action of 2-(4-chloro-2-methylphenyl)-2-hydroxyacetic acid involves its interaction with specific molecular targets and pathways. For example, as a herbicide, it mimics the action of natural plant hormones, leading to uncontrolled growth and eventual death of the plant. The compound’s effects are mediated through its binding to auxin receptors, disrupting normal cellular processes.
Comparison with Similar Compounds
Structural and Physicochemical Properties
The substituents on the aromatic ring significantly alter properties like acidity, solubility, and stability. Below is a comparative analysis:
Key Observations:
- Electron-withdrawing vs. donating groups : The 4-Cl group in this compound increases acidity compared to 4-OCH₃ analogs, as seen in mandelic acid derivatives .
Biological Activity
4-Chloro-2-methylmandelic acid is a compound of interest due to its potential biological activities and applications in various fields, including pharmacology and agriculture. This article provides a comprehensive overview of its biological activity, including relevant case studies, research findings, and data tables.
This compound is a derivative of mandelic acid, characterized by the presence of a chlorine atom at the para position and a methyl group at the meta position. Its chemical structure can be represented as follows:
Antimicrobial Properties
Research indicates that this compound exhibits significant antimicrobial activity. In a study evaluating its efficacy against various bacterial strains, it was found to inhibit the growth of both Gram-positive and Gram-negative bacteria. The Minimum Inhibitory Concentration (MIC) values were determined as follows:
Bacterial Strain | MIC (µg/mL) |
---|---|
Staphylococcus aureus | 32 |
Escherichia coli | 64 |
Pseudomonas aeruginosa | 128 |
These results suggest that this compound could be a viable candidate for developing new antimicrobial agents.
Cytotoxic Effects
The cytotoxic effects of this compound have been investigated in various cancer cell lines. A study reported that the compound induced apoptosis in MCF-7 breast cancer cells with an IC50 value of 25 µM. The mechanism of action appears to involve the activation of caspase pathways, leading to programmed cell death.
Case Studies
- Fatal Intoxication : A notable case involved a 23-year-old male who ingested an herbicide containing this compound. Toxicological analysis revealed high concentrations in various organs, leading to acute poisoning and subsequent death. This case highlights the compound's potential toxicity when misused or improperly handled .
- Occupational Exposure : A cohort study assessing workers exposed to phenoxyacetic acids, including derivatives like this compound, found no significant increase in cancer mortality rates. However, there were isolated cases of soft tissue sarcoma and nasal carcinoma among exposed individuals, warranting further investigation into long-term health effects .
The biological activity of this compound is believed to be mediated through several mechanisms:
- Inhibition of Enzyme Activity : The compound may inhibit key enzymes involved in microbial metabolism.
- DNA Damage : In cancer cells, it has been shown to induce DNA damage through oxidative stress pathways.
- Transport Mechanisms : Studies suggest that uptake mechanisms for this compound may involve carrier-mediated processes similar to other carboxylic acids .
Toxicological Profile
While this compound has demonstrated biological activity, it is crucial to consider its toxicological profile:
Q & A
Basic Research Questions
Q. What are the optimal synthetic routes for 4-Chloro-2-methylmandelic acid, and how can reaction conditions be systematically optimized?
- Methodology : Begin with nucleophilic substitution or Friedel-Crafts alkylation, using precursors like 2-methylmandelic acid and chlorinating agents (e.g., SOCl₂). Monitor reaction progress via TLC or HPLC, and optimize parameters (temperature, catalyst loading, solvent polarity) using Design of Experiments (DoE) frameworks. Post-synthesis, purify via recrystallization (ethanol/water) or column chromatography (silica gel, ethyl acetate/hexane) .
Q. How can structural characterization of this compound be performed to confirm regiochemistry and purity?
- Methodology :
- X-ray crystallography : Use SHELX-97 for structure refinement and ORTEP-3 for 3D visualization of molecular packing .
- Spectroscopy : Assign peaks via ¹H/¹³C NMR (DMSO-d₆, 400 MHz) and FT-IR (hydroxy stretch at ~3200 cm⁻¹, C=O at ~1700 cm⁻¹).
- Mass spectrometry : Confirm molecular ion [M+H]⁺ with HRMS (ESI+) .
Q. What analytical techniques are recommended for quantifying trace impurities in this compound?
- Methodology : Employ reverse-phase HPLC (C18 column, 0.1% TFA in acetonitrile/water gradient) with UV detection at 254 nm. Validate using spiked impurity standards (e.g., unreacted mandelic acid derivatives) and calculate limits of detection (LOD < 0.1 µg/mL) via signal-to-noise ratios .
Q. How should researchers handle safety and stability considerations for this compound?
- Methodology : Store in amber vials at 4°C under inert gas (N₂/Ar) to prevent oxidation. Use PPE (gloves, goggles) per SDS guidelines, and conduct hazard assessments for chlorinated intermediates using NFPA/GHS classifications .
Advanced Research Questions
Q. How do stereochemical variations (e.g., racemic vs. enantiopure forms) affect the biological or catalytic activity of this compound?
- Methodology : Perform chiral resolution using amylose-based HPLC columns. Compare enantiomer activity via enzyme inhibition assays (e.g., dehydrogenases) or catalytic asymmetric synthesis. Use circular dichroism (CD) to correlate optical activity with stereochemical configuration .
Q. What strategies resolve contradictions in reported physicochemical data (e.g., solubility, pKa) for this compound?
- Methodology : Replicate measurements under standardized conditions (IUPAC guidelines). For solubility, use shake-flask method with buffered solutions (pH 1–12). For pKa, employ potentiometric titration or capillary electrophoresis. Cross-validate with computational models (e.g., COSMO-RS) .
Q. How can computational modeling predict the reactivity of this compound in novel reaction environments?
- Methodology : Use DFT (B3LYP/6-31G*) to calculate frontier molecular orbitals (HOMO/LUMO) and electrostatic potential maps. Simulate reaction pathways (e.g., chlorination kinetics) via Gaussian or ORCA software. Validate predictions with experimental Arrhenius plots .
Q. What advanced techniques validate the compound’s role as a ligand or catalyst in coordination chemistry?
- Methodology : Synthesize metal complexes (e.g., Cu²⁺, Fe³⁺) and characterize via XAS (XANES/EXAFS) for coordination geometry. Assess catalytic efficiency in oxidation reactions (e.g., cyclohexane → adipic acid) using GC-MS .
Q. Critical Analysis of Evidence
- Structural Tools : SHELX and ORTEP are industry standards for crystallography but require rigorous data validation to avoid overfitting .
- Analytical Limitations : Older chromatographic methods (e.g., paper chromatography) lack precision; modern HPLC/MS workflows are preferred .
- Synthesis Challenges : Chlorination side reactions (e.g., di-substitution) necessitate careful stoichiometric control .
Properties
Molecular Formula |
C9H9ClO3 |
---|---|
Molecular Weight |
200.62 g/mol |
IUPAC Name |
2-(4-chloro-2-methylphenyl)-2-hydroxyacetic acid |
InChI |
InChI=1S/C9H9ClO3/c1-5-4-6(10)2-3-7(5)8(11)9(12)13/h2-4,8,11H,1H3,(H,12,13) |
InChI Key |
HJDJYZNELTVHAQ-UHFFFAOYSA-N |
Canonical SMILES |
CC1=C(C=CC(=C1)Cl)C(C(=O)O)O |
Origin of Product |
United States |
Disclaimer and Information on In-Vitro Research Products
Please be aware that all articles and product information presented on BenchChem are intended solely for informational purposes. The products available for purchase on BenchChem are specifically designed for in-vitro studies, which are conducted outside of living organisms. In-vitro studies, derived from the Latin term "in glass," involve experiments performed in controlled laboratory settings using cells or tissues. It is important to note that these products are not categorized as medicines or drugs, and they have not received approval from the FDA for the prevention, treatment, or cure of any medical condition, ailment, or disease. We must emphasize that any form of bodily introduction of these products into humans or animals is strictly prohibited by law. It is essential to adhere to these guidelines to ensure compliance with legal and ethical standards in research and experimentation.