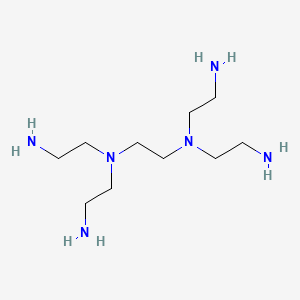
1,2-Ethanediamine, N,N,N',N'-tetrakis(2-aminoethyl)-
- Click on QUICK INQUIRY to receive a quote from our team of experts.
- With the quality product at a COMPETITIVE price, you can focus more on your research.
Overview
Description
1,2-Ethanediamine, N,N,N’,N’-tetrakis(2-aminoethyl)-, also known as TETA, is a chemical compound with the molecular formula C8H24N4 . It is a clear, colorless liquid with a strong ammonia-like odor and is water-soluble .
Molecular Structure Analysis
The molecular structure of 1,2-Ethanediamine, N,N,N’,N’-tetrakis(2-aminoethyl)- is based on its molecular formula, C8H24N4 . The exact structure can be viewed using specialized chemical structure viewing software .Physical And Chemical Properties Analysis
1,2-Ethanediamine, N,N,N’,N’-tetrakis(2-aminoethyl)- is a clear, colorless liquid with a strong ammonia-like odor . It is water-soluble . More detailed physical and chemical properties, such as boiling point and density, were not found in the sources.Scientific Research Applications
1. Complex Formation and Spectral Studies
1,2-Ethanediamine, N,N,N',N'-tetrakis(2-aminoethyl) is used in the preparation of various metal complexes. For example, it forms sexidentate ligands with cobalt(III) in different configurations, and these complexes have been studied for their electronic spectra characteristics. Such studies provide valuable insights into ligand behavior and metal-ligand interactions (Hata, Doh, Kashiwabara, & Fujita, 1981).
2. Synthesis of Hexadentate Ligands and Crystal Structures
This compound also plays a crucial role in the synthesis of hexadentate ligands like N,N,N',N'-tetrakis-(2-benzimidazolylmethyl)-1,2-ethanediamine, which have been used to form allomeric complexes with various metal ions. The study of these complexes includes elemental analysis and X-ray structural analysis, contributing to the understanding of crystal architecture in coordination chemistry (Tong, Wu, Tian, & Yan, 2012).
3. Applications in Synthesis and Catalysis
The compound is also essential in synthesizing various derivatives that are used in different chemical reactions. For instance, its derivatives have been used as catalysts in alkene epoxidation, showcasing its potential in catalytic processes (Jiang, Gorden, & Goldsmith, 2013).
4. Studies in Metal Complexes and Hydrolyses
Research involving this compound extends to studying the stability and hydrolysis of metal complexes. Such studies are crucial in inorganic chemistry and can contribute to understanding metal ion behavior in different chemical environments (Curtis, Hendry, & Lawrance, 1988).
Mechanism of Action
Target of Action
Similar compounds are often used as chelating agents, suggesting that their targets could be metal ions .
Mode of Action
The compound’s mode of action is likely related to its ability to form stable complexes with metal ions . This interaction could result in changes to the metal ions’ reactivity or solubility, potentially affecting various biochemical processes.
properties
IUPAC Name |
N'-(2-aminoethyl)-N'-[2-[bis(2-aminoethyl)amino]ethyl]ethane-1,2-diamine |
Source
|
---|---|---|
Source | PubChem | |
URL | https://pubchem.ncbi.nlm.nih.gov | |
Description | Data deposited in or computed by PubChem | |
InChI |
InChI=1S/C10H28N6/c11-1-5-15(6-2-12)9-10-16(7-3-13)8-4-14/h1-14H2 |
Source
|
Source | PubChem | |
URL | https://pubchem.ncbi.nlm.nih.gov | |
Description | Data deposited in or computed by PubChem | |
InChI Key |
BPFBNAXGHSFGDC-UHFFFAOYSA-N |
Source
|
Source | PubChem | |
URL | https://pubchem.ncbi.nlm.nih.gov | |
Description | Data deposited in or computed by PubChem | |
Canonical SMILES |
C(CN(CCN)CCN(CCN)CCN)N |
Source
|
Source | PubChem | |
URL | https://pubchem.ncbi.nlm.nih.gov | |
Description | Data deposited in or computed by PubChem | |
Molecular Formula |
C10H28N6 |
Source
|
Source | PubChem | |
URL | https://pubchem.ncbi.nlm.nih.gov | |
Description | Data deposited in or computed by PubChem | |
DSSTOX Substance ID |
DTXSID40444404 |
Source
|
Record name | 1,2-Ethanediamine, N,N,N',N'-tetrakis(2-aminoethyl)- | |
Source | EPA DSSTox | |
URL | https://comptox.epa.gov/dashboard/DTXSID40444404 | |
Description | DSSTox provides a high quality public chemistry resource for supporting improved predictive toxicology. | |
Molecular Weight |
232.37 g/mol |
Source
|
Source | PubChem | |
URL | https://pubchem.ncbi.nlm.nih.gov | |
Description | Data deposited in or computed by PubChem | |
Product Name |
1,2-Ethanediamine, N,N,N',N'-tetrakis(2-aminoethyl)- | |
CAS RN |
4097-90-9 |
Source
|
Record name | 1,2-Ethanediamine, N,N,N',N'-tetrakis(2-aminoethyl)- | |
Source | EPA DSSTox | |
URL | https://comptox.epa.gov/dashboard/DTXSID40444404 | |
Description | DSSTox provides a high quality public chemistry resource for supporting improved predictive toxicology. | |
Disclaimer and Information on In-Vitro Research Products
Please be aware that all articles and product information presented on BenchChem are intended solely for informational purposes. The products available for purchase on BenchChem are specifically designed for in-vitro studies, which are conducted outside of living organisms. In-vitro studies, derived from the Latin term "in glass," involve experiments performed in controlled laboratory settings using cells or tissues. It is important to note that these products are not categorized as medicines or drugs, and they have not received approval from the FDA for the prevention, treatment, or cure of any medical condition, ailment, or disease. We must emphasize that any form of bodily introduction of these products into humans or animals is strictly prohibited by law. It is essential to adhere to these guidelines to ensure compliance with legal and ethical standards in research and experimentation.