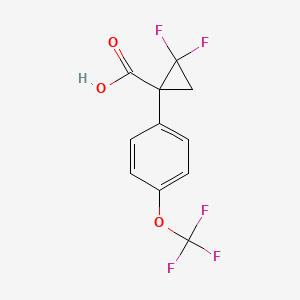
22-Difluoro-1-(4-(trifluoromethoxy)phenyl)cyclopropane-1-carboxylic acid
- Click on QUICK INQUIRY to receive a quote from our team of experts.
- With the quality product at a COMPETITIVE price, you can focus more on your research.
Overview
Description
22-Difluoro-1-(4-(trifluoromethoxy)phenyl)cyclopropane-1-carboxylic acid is a fluorinated cyclopropane derivative characterized by a strained three-membered cyclopropane ring substituted with two fluorine atoms, a trifluoromethoxy-phenyl group, and a carboxylic acid moiety. The cyclopropane ring imparts significant steric strain, which can enhance reactivity or influence conformational stability in biological systems .
Preparation Methods
Synthetic Routes and Reaction Conditions: One common method includes the use of a cyclopropane ring formation reaction, such as the Simmons-Smith reaction, followed by fluorination using reagents like diethylaminosulfur trifluoride (DAST) or similar fluorinating agents .
Industrial Production Methods: Industrial production of this compound may involve large-scale cyclopropanation and fluorination processes, utilizing continuous flow reactors to ensure efficient and controlled synthesis. The use of advanced fluorination techniques and catalysts can enhance yield and purity, making the process economically viable for large-scale production .
Chemical Reactions Analysis
Esterification
The carboxylic acid group undergoes esterification with alcohols or other nucleophiles to form esters. This reaction is fundamental in organic synthesis and is facilitated by the acidic nature of the carboxylic proton. The trifluoromethoxy group on the phenyl ring enhances the electron-withdrawing effect, potentially stabilizing the transition state during ester formation.
Deoxyfluorination
The compound participates in deoxyfluorination reactions, converting the carboxylic acid into acyl fluorides. This process typically employs reagents like pentafluoropyridine (PFP), which acts as both a fluoride source and an activating agent. The reaction proceeds via nucleophilic aromatic substitution (S_NAr) mechanisms, where the electron-deficient carboxylate attacks PFP. For example:
-
Mechanism :
Amide Bond Formation
The compound can form amides through one-pot deoxyfluorination reactions. In this process:
-
Acyl fluoride generation : The carboxylic acid is converted to an acyl fluoride using PFP.
-
Amine coupling : The acyl fluoride reacts with amines (e.g., benzylamine) to form amides.
This method avoids the need for isolating intermediates, simplifying synthesis workflows .
Nucleophilic Aromatic Substitution (S_NAr)
The trifluoromethoxy group on the phenyl ring directs reactivity toward nucleophilic aromatic substitution. The electron-withdrawing effect of the trifluoromethoxy group activates adjacent positions for nucleophilic attack. This reactivity is critical in medicinal chemistry for modifying aromatic systems.
Cyclopropane Ring Reactions
The cyclopropane ring undergoes ring-opening reactions under specific conditions (e.g., base or acid catalysis). These reactions generate substituted alkenes or other intermediates, which can be utilized in further synthetic steps. For example:
Electrophilic Aromatic Substitution
Despite the electron-withdrawing trifluoromethoxy group, the phenyl ring may participate in electrophilic aromatic substitution at positions ortho or para to the substituent. This reactivity is influenced by the directing effects of the trifluoromethoxy group.
Reaction Comparison Table
Reaction Type | Key Features | Mechanism | Applications |
---|---|---|---|
Esterification | Reacts with alcohols to form esters. | Acid-catalyzed nucleophilic substitution | Pharmaceutical synthesis, prodrugs |
Deoxyfluorination | Converts carboxylic acid to acyl fluoride using PFP. | S_NAr mechanism, fluoride displacement | Amide synthesis, peptide chemistry |
Amide Bond Formation | One-pot reaction with amines via acyl fluoride intermediates. | Sequential deoxyfluorination and coupling | Drug development, materials science |
S_NAr | Activated by trifluoromethoxy group; directs nucleophilic attack. | Electron-deficient aromatic substitution | Functionalization of aromatic systems |
Cyclopropane Ring Opening | Generates alkenes/alkynes under base or acid conditions. | Strained ring opening via base/acid | Organic synthesis intermediates |
Research Findings
-
Fluorine Effects : The trifluoromethoxy and difluoro groups enhance lipophilicity and stability, critical for biological applications.
-
Mechanistic Insights : Deoxyfluorination with PFP involves intermediate acyl fluoride formation, confirmed by 19F NMR and LCMS analysis .
-
Synthetic Utility : The compound’s reactivity enables its use in medicinal chemistry (e.g., anti-inflammatory agents) and agrochemicals.
Scientific Research Applications
Chemistry: The compound is used as a building block in organic synthesis, particularly in the development of fluorinated pharmaceuticals and agrochemicals.
Biology: In biological research, the compound is studied for its potential as a fluorinated probe in imaging and diagnostic applications. The presence of fluorine atoms can enhance the compound’s interaction with biological targets, making it useful in studying enzyme mechanisms and protein-ligand interactions .
Medicine: The compound’s stability and reactivity make it a candidate for drug development, particularly in designing molecules with improved pharmacokinetic properties. Fluorinated compounds often exhibit enhanced metabolic stability and reduced toxicity, making them attractive for medicinal chemistry .
Industry: In the industrial sector, the compound is used in the development of advanced materials, such as fluorinated polymers and coatings. Its chemical stability and resistance to degradation make it suitable for applications in harsh environments .
Mechanism of Action
The mechanism by which 2,2-difluoro-1-[4-(trifluoromethoxy)phenyl]cyclopropane-1-carboxylic acid exerts its effects involves its interaction with molecular targets through its fluorine atoms. The electronegativity of fluorine enhances the compound’s binding affinity to specific enzymes or receptors, potentially inhibiting or modulating their activity. The compound’s unique structure allows it to participate in various biochemical pathways, influencing cellular processes and metabolic functions .
Comparison with Similar Compounds
Comparison with Structurally Similar Compounds
The following analysis compares the target compound with analogs from the provided evidence, focusing on structural features, physicochemical properties, and inferred applications.
1-(4-Chlorophenyl)-1-cyclobutanecarboxylic Acid (CAS RN 50921-39-6)
- Structure : Cyclobutane ring (four-membered) with a 4-chlorophenyl substituent and carboxylic acid.
- Molecular Weight : 210.65 g/mol.
- Melting Point : 80–82°C.
- Chlorine substituent (-Cl) is less electronegative and lipophilic than trifluoromethoxy (-OCF₃), which may reduce bioavailability in biological systems. Applications: Catalogued as a reagent (Kanto Reagents), suggesting laboratory use rather than direct agrochemical/pharmaceutical roles .
Cyclanilide (1-(((2,4-dichlorophenyl)amino)carbonyl)cyclopropanecarboxylic Acid)
- Structure : Cyclopropane ring with a urea-linked 2,4-dichlorophenyl group and carboxylic acid.
- Key Differences :
- The urea functional group (-NH-C(=O)-NH-) introduces hydrogen-bonding capacity, enhancing solubility in polar solvents compared to the target compound’s trifluoromethoxy group.
- Dichlorophenyl substituent (-C₆H₃Cl₂) provides moderate electronegativity but lacks the steric bulk of -OCF₃.
- Applications: Registered as a herbicide, indicating that cyclopropane carboxylic acids with aryl substituents are viable agrochemicals .
Trifluoromethoxy-Substituted Benzoic Acid Esters (e.g., [288579-85-1])
- Structure : Benzoic acid esterified with a 4-(trifluoromethoxy)phenyl group.
- Esterification replaces the carboxylic acid’s acidity with a more hydrolytically stable ester group, affecting solubility and reactivity. Applications: Perfluorinated compounds like these are often used in surfactants or liquid crystals due to their stability and hydrophobic properties .
Data Table: Comparative Analysis of Key Compounds
Research Findings and Implications
- Ring Strain and Reactivity : The cyclopropane ring in the target compound likely increases reactivity compared to cyclobutane analogs (e.g., 1-(4-chlorophenyl)-1-cyclobutanecarboxylic acid), making it more suitable for covalent binding in enzyme inhibition .
- Fluorination Effects : The trifluoromethoxy group enhances lipophilicity (logP ~3.5 estimated) compared to chlorine or urea groups, which could improve blood-brain barrier penetration in pharmaceutical contexts .
- Biological Activity : Cyclopropane carboxylic acids with electron-withdrawing substituents (e.g., -F, -OCF₃) are associated with prolonged herbicidal activity due to resistance to oxidative degradation .
Biological Activity
22-Difluoro-1-(4-(trifluoromethoxy)phenyl)cyclopropane-1-carboxylic acid (CAS: 2229533-86-0) is a novel compound that has garnered attention for its potential biological activities, particularly in the field of plant biochemistry and medicinal chemistry. This article presents a comprehensive overview of its biological activity, including synthesis, structure-activity relationships, and relevant case studies.
The compound features a cyclopropane ring substituted with difluoro and trifluoromethoxy groups, which are known to influence its biological properties. The molecular formula is C11H8F5O2, and its structure can be represented as follows:
Synthesis
The synthesis of this compound typically involves multi-step organic reactions, including the formation of the cyclopropane ring via cyclopropanation methods, followed by functional group modifications to introduce the difluoro and trifluoromethoxy substituents. Recent studies have utilized copper(I) salt/amine/DMSO systems to enhance yields under mild conditions .
Ethylene Biosynthesis Inhibition
One of the significant biological activities attributed to this compound is its ability to inhibit ethylene biosynthesis in plants. Ethylene is a crucial plant hormone involved in various physiological processes, including fruit ripening and stress responses. The compound acts as an inhibitor of 1-aminocyclopropane-1-carboxylate oxidase (ACO), an enzyme critical for ethylene production in plants like Arabidopsis thaliana.
Table 1: Binding Affinity and Inhibition Potency
Compound | ΔG (kcal/mol) | Kb (M−1) |
---|---|---|
This compound | -6.5 | 5.9385 × 10^4 |
Methylcyclopropane | -3.1 | 0.188 × 10^3 |
Pyrazinoic acid | -5.3 | 7.61 × 10^3 |
The docking studies indicate that the compound exhibits a favorable binding affinity towards the ACO enzyme, suggesting its potential as a bioregulator in agricultural applications .
Anticancer Activity
In addition to its role in plant biology, preliminary assessments indicate that derivatives of cyclopropane carboxylic acids, including this compound, may possess anticancer properties. Studies have shown that structurally similar compounds exhibit effective inhibition of cell proliferation in human leukemia cell lines without significant cytotoxicity .
Case Study: Structure-Activity Relationship (SAR)
A series of derivatives were synthesized to evaluate their anticancer activity against U937 human myeloid leukemia cells. The results demonstrated that modifications to the cyclopropane core significantly influenced biological activity, with certain substitutions enhancing inhibitory effects on cell growth while maintaining low toxicity levels .
Q & A
Basic Research Questions
Q. What are the recommended synthetic routes for preparing 22-difluoro-1-(4-(trifluoromethoxy)phenyl)cyclopropane-1-carboxylic acid?
- Methodological Answer : The synthesis typically involves a [2+1] cyclopropanation strategy. A difluorocarbene source (e.g., sodium chlorodifluoroacetate) reacts with a styrene derivative containing the 4-(trifluoromethoxy)phenyl group to form the cyclopropane ring. Post-functionalization includes hydrolysis of ester intermediates to yield the carboxylic acid. For example, similar cyclopropane derivatives (e.g., 2,2-difluorocyclopropanecarboxylic acid) are synthesized via carbene insertion, with purification by recrystallization (mp 124–126°C observed in analogs) .
Q. How can researchers ensure purity and structural fidelity during synthesis?
- Methodological Answer : Purity is assessed using HPLC (reverse-phase C18 columns, acetonitrile/water mobile phase) and 19F NMR (to confirm fluorination patterns). Melting point analysis (e.g., mp 110–114°C for structurally related compounds) provides preliminary purity validation . For chiral purity, chiral stationary phase HPLC or circular dichroism is recommended if stereocenters are present .
Q. What analytical techniques are critical for characterizing this compound?
- Methodological Answer :
- 19F NMR : Resolves trifluoromethoxy (-OCF₃) and difluorocyclopropane signals, with chemical shifts typically between -55 to -65 ppm for CF₃ groups.
- HRMS (High-Resolution Mass Spectrometry) : Confirms molecular formula (e.g., C₁₁H₈F₅O₃).
- X-ray crystallography : Resolves cyclopropane ring geometry and substituent orientation, critical for structure-activity studies .
Q. How does the compound’s stability vary under different storage conditions?
- Methodological Answer : Stability studies should include:
- Thermal stability : TGA/DSC analysis (decomposition >200°C inferred from analogs).
- Hydrolytic stability : Incubate in buffers (pH 1–13) at 37°C for 24–72 hours, monitored by HPLC. The electron-withdrawing trifluoromethoxy group may enhance resistance to hydrolysis compared to non-fluorinated analogs .
Advanced Research Questions
Q. How can researchers address cis/trans isomerism in the cyclopropane ring?
- Methodological Answer : Isomer separation is achieved via preparative HPLC (e.g., using CHIRALPAK® IG columns) or crystallization in polar solvents (e.g., ethanol/water). DFT calculations (B3LYP/6-31G*) predict relative stability: trans-isomers often dominate due to reduced steric clash between the trifluoromethoxy phenyl group and carboxylic acid .
Q. What strategies optimize catalytic functionalization of the cyclopropane core?
- Methodological Answer :
- Cross-coupling : Suzuki-Miyaura reactions with aryl boronic acids to modify the phenyl ring. Use Pd(PPh₃)₄ catalyst and Cs₂CO₃ base in THF/H₂O.
- Decarboxylation : Thermal or photolytic decarboxylation (e.g., 200°C in DMF) yields difluorocyclopropane derivatives for further reactivity studies .
Q. How can computational modeling predict the compound’s reactivity in biological systems?
- Methodological Answer :
- DFT calculations : Evaluate frontier molecular orbitals (HOMO/LUMO) to predict electrophilic/nucleophilic sites. The carboxylic acid group acts as a hydrogen-bond donor, while the CF₃O group enhances lipid solubility (logP ~2.5 calculated via ChemAxon).
- MD simulations : Model interactions with lipid bilayers or protein targets (e.g., cyclooxygenase enzymes) to guide drug design .
Q. What are the challenges in reconciling contradictory spectroscopic data across studies?
- Methodological Answer : Contradictions in 19F NMR shifts or melting points may arise from:
- Solvent effects : Deuterated solvents (CDCl₃ vs. DMSO-d₆) alter chemical shifts.
- Impurity profiles : Trace isomers or residual catalysts (e.g., Pd in cross-coupled products) skew data. Validate via spiking experiments with authentic standards .
Q. How can metabolite identification studies be designed for this compound?
- Methodological Answer :
- In vitro assays : Incubate with liver microsomes (human/rat) and NADPH cofactor. Quench with acetonitrile, extract metabolites, and analyze via LC-MS/MS (Q-TOF preferred for high resolution).
- Phase I metabolites : Look for hydroxylation at the cyclopropane ring or O-demethylation of the trifluoromethoxy group .
Properties
Molecular Formula |
C11H7F5O3 |
---|---|
Molecular Weight |
282.16 g/mol |
IUPAC Name |
2,2-difluoro-1-[4-(trifluoromethoxy)phenyl]cyclopropane-1-carboxylic acid |
InChI |
InChI=1S/C11H7F5O3/c12-10(13)5-9(10,8(17)18)6-1-3-7(4-2-6)19-11(14,15)16/h1-4H,5H2,(H,17,18) |
InChI Key |
CCFGYKUWJUXGIQ-UHFFFAOYSA-N |
Canonical SMILES |
C1C(C1(F)F)(C2=CC=C(C=C2)OC(F)(F)F)C(=O)O |
Origin of Product |
United States |
Disclaimer and Information on In-Vitro Research Products
Please be aware that all articles and product information presented on BenchChem are intended solely for informational purposes. The products available for purchase on BenchChem are specifically designed for in-vitro studies, which are conducted outside of living organisms. In-vitro studies, derived from the Latin term "in glass," involve experiments performed in controlled laboratory settings using cells or tissues. It is important to note that these products are not categorized as medicines or drugs, and they have not received approval from the FDA for the prevention, treatment, or cure of any medical condition, ailment, or disease. We must emphasize that any form of bodily introduction of these products into humans or animals is strictly prohibited by law. It is essential to adhere to these guidelines to ensure compliance with legal and ethical standards in research and experimentation.