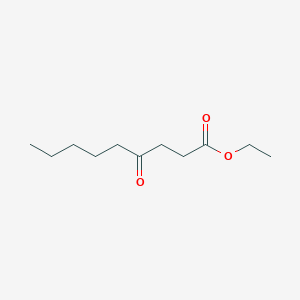
Ethyl 4-oxononanoate
Overview
Description
Ethyl 4-oxononanoate (CAS: 37174-96-2) is a keto-ester compound with the molecular formula C₁₁H₂₀O₃ and a molecular weight of 200.28 g/mol . Structurally, it consists of a nine-carbon aliphatic chain with a ketone group at the fourth position and an ethyl ester moiety at the terminal carboxyl group. This compound is primarily synthesized via ozonolysis of 6-methylhept-5-en-2-one, followed by esterification, as reported in asymmetric synthesis protocols for tropinone derivatives and 1,3-amino alcohols . Its key applications include serving as a precursor for ketal-protected esters, which are intermediates in organic synthesis .
Preparation Methods
Synthetic Routes and Reaction Conditions
Ethyl 4-oxononanoate can be synthesized through several methods. One common synthetic route involves the esterification of 4-oxononanoic acid with ethanol in the presence of a strong acid catalyst such as sulfuric acid. The reaction is typically carried out under reflux conditions to ensure complete conversion of the acid to the ester .
Industrial Production Methods
In industrial settings, the production of this compound often involves the use of continuous flow reactors to optimize reaction conditions and increase yield. The process may include steps such as purification through distillation and crystallization to obtain the desired purity level .
Chemical Reactions Analysis
Types of Reactions
Ethyl 4-oxononanoate undergoes various chemical reactions, including:
Oxidation: It can be oxidized to form corresponding carboxylic acids.
Reduction: It can be reduced to form alcohols.
Substitution: It can undergo nucleophilic substitution reactions to form different esters or amides.
Common Reagents and Conditions
Oxidation: Common oxidizing agents include potassium permanganate and chromium trioxide.
Reduction: Reducing agents such as lithium aluminum hydride or sodium borohydride are typically used.
Substitution: Nucleophiles such as amines or alcohols can be used under acidic or basic conditions.
Major Products Formed
Oxidation: 4-oxononanoic acid.
Reduction: 4-hydroxynonanoate.
Substitution: Various esters or amides depending on the nucleophile used.
Scientific Research Applications
Scientific Research Applications
1. Chemistry:
- Intermediate in Organic Synthesis: Ethyl 4-oxononanoate serves as an important intermediate in the synthesis of more complex organic molecules. It can participate in various reactions to form derivatives that are useful in pharmaceuticals and agrochemicals .
2. Biology:
- Biological Activity Studies: Research has indicated that this compound may exhibit biological activities, including interactions with enzymes. Its potential role as a substrate for specific enzymes suggests avenues for further exploration in enzymology and metabolic studies.
3. Medicine:
- Therapeutic Potential: The compound is being investigated for its therapeutic properties, particularly as a precursor in drug synthesis. Its structural features may contribute to the development of new pharmaceutical agents targeting various diseases .
4. Industrial Applications:
- Production of Fragrances and Flavors: this compound is utilized in the production of fragrances and flavors due to its pleasant aroma profile. It finds applications in the cosmetic and food industries, enhancing product appeal .
Data Tables
Case Studies
- Synthesis of Bioactive Compounds:
- Enzymatic Studies:
-
Industrial Production Methods:
- A case study on industrial production methods revealed that continuous flow reactors could optimize the synthesis of this compound, enhancing yield and purity levels. This approach demonstrates its feasibility for large-scale applications in fragrance production.
Mechanism of Action
The mechanism of action of ethyl 4-oxononanoate involves its interaction with specific molecular targets and pathways. For example, it may act as a substrate for certain enzymes, leading to the formation of biologically active metabolites. The exact pathways and targets can vary depending on the specific application and context of use .
Comparison with Similar Compounds
Structural and Functional Analogues
The following table summarizes key structural analogs of ethyl 4-oxononanoate, highlighting differences in molecular properties, synthesis, and applications:
Key Observations
Functional Group Influence: Ethyl and methyl esters differ in chain length and lipophilicity. This compound’s longer chain (C₁₁) enhances steric bulk compared to methyl analogs (C₁₀) .
Synthesis Methods: this compound is synthesized via ozonolysis, a method favoring controlled oxidation . Methyl derivatives (5v, 5r, 5t) employ visible-light-mediated bromine radical reactions, enabling efficient C–H functionalization under mild conditions .
Spectroscopic Data: ¹³C NMR Shifts: this compound’s ketone carbonyl resonates at δ 208.92 ppm, while methyl analogs show upfield shifts (e.g., δ 209.11 ppm for 5t) due to electronic effects of substituents . C₁₈H₂₅ClO₃ formula) suggest possible nomenclature errors in source materials .
Applications: this compound is critical in asymmetric synthesis, whereas methyl derivatives are intermediates for flavonoids and bioactive molecules . Ethyl 6-oxohexanoate () demonstrates how chain length and heterocyclic substituents (thiomorpholine) expand utility in drug discovery .
Biological Activity
Ethyl 4-oxononanoate, a compound with the molecular formula C₁₁H₂₄O₃, has garnered attention in scientific research for its potential biological activities and applications across various fields, including biochemistry, pharmacology, and industrial chemistry. This article explores the biological activity of this compound, highlighting its mechanisms of action, relevant case studies, and research findings.
This compound is characterized by a carbonyl group adjacent to an ethyl ester functional group, which contributes to its reactivity and biological properties. The compound is studied for its interactions with enzymes and cellular pathways, suggesting potential therapeutic applications. It may act as a substrate for specific enzymes, leading to the formation of biologically active metabolites. This interaction is crucial in understanding its role in metabolic processes and potential therapeutic effects.
1. Enzyme Interactions
Research indicates that this compound may interact with various enzymes, affecting metabolic pathways. For instance, it has been observed to influence the activity of mitochondrial enzymes, potentially altering energy metabolism and oxidative stress responses in cells .
2. Antioxidant Properties
The compound exhibits antioxidant properties that may help mitigate oxidative stress. Similar compounds like 4-oxo-2-nonenal (4-ONE) have been shown to impact mitochondrial function and oxidative damage. This compound's structural similarities suggest it may also possess protective effects against oxidative damage in cellular systems .
Case Study: Mitochondrial Function
A study investigating the effects of lipid peroxidation products on bovine heart mitochondria revealed that compounds similar to this compound could significantly alter mitochondrial structure and function. The study demonstrated that these compounds could inhibit mitochondrial antioxidant systems, leading to increased oxidative stress and cellular damage .
Table 1: Effects on Mitochondrial Parameters
Parameter | Control (Ethanol) | 4-HNE (1 mM) | 4-ONE (1 mM) |
---|---|---|---|
Oxygen Consumption Rate (OCR) | Baseline | Decreased | Significantly Decreased |
Mitochondrial Permeability | Normal | Increased | Increased |
Morphological Changes | Normal | Altered | More Altered |
The results indicate that while both 4-HNE and 4-ONE affect mitochondrial function, the latter exhibits a more pronounced impact on mitochondrial integrity and function .
3. Pharmacological Applications
This compound is being explored for potential therapeutic applications. Its ability to modulate enzyme activity suggests it could be developed as a precursor in drug synthesis or as a therapeutic agent targeting specific metabolic pathways .
Q & A
Basic Research Questions
Q. How can researchers identify and characterize Ethyl 4-oxononanoate in a mixture of esters?
- Methodological Answer : this compound can be distinguished using gas chromatography-mass spectrometry (GC-MS) coupled with retention index matching. The compound’s molecular weight (200.28 g/mol) and CAS number (276.30) from validated databases like SciFinder or Reaxys should be cross-referenced . Nuclear magnetic resonance (NMR) spectroscopy, particularly H and C NMR, can confirm structural features such as the ethyl ester group and ketone functionality. For example, the ketone proton at δ 2.4–2.6 ppm and the ethyl group’s triplet at δ 1.2–1.3 ppm are diagnostic .
Q. What are the standard protocols for synthesizing this compound?
- Methodological Answer : A common approach involves the Claisen condensation of ethyl acetoacetate with hexanoic acid derivatives under basic conditions. Reaction optimization requires monitoring pH (8–10) and temperature (60–80°C) to minimize side products like β-keto ester degradation. Post-synthesis purification via fractional distillation or column chromatography (silica gel, hexane/ethyl acetate eluent) ensures ≥95% purity .
Q. How can researchers assess the purity of this compound for kinetic studies?
- Methodological Answer : Purity is validated using high-performance liquid chromatography (HPLC) with a C18 column and UV detection at 210 nm. Calibrate against a certified reference standard (CRS) from authoritative sources like NIST. Discrepancies in melting points or optical rotation values compared to literature (e.g., NIST Chemistry WebBook) indicate impurities requiring recrystallization .
Advanced Research Questions
Q. What experimental designs are optimal for studying this compound’s reactivity in catalytic hydrogenation?
- Methodological Answer : Use a controlled-pressure hydrogenation reactor with palladium-on-carbon (Pd/C) or Raney nickel catalysts. Independent variables include catalyst loading (1–5 wt%), hydrogen pressure (1–5 atm), and temperature (25–80°C). Monitor reaction progress via in-situ IR spectroscopy to track ketone reduction (disappearance of C=O stretch at ~1700 cm). Statistical tools like ANOVA should analyze yield variations across trials .
Q. How should researchers resolve contradictory data in this compound’s thermal stability studies?
- Methodological Answer : Discrepancies in thermogravimetric analysis (TGA) results may arise from moisture content or heating rate inconsistencies. Replicate experiments under inert atmospheres (N or Ar) with controlled humidity (<5% RH). Compare decomposition onset temperatures (T) across multiple trials and apply error bars (±2°C) to assess significance. Cross-validate with differential scanning calorimetry (DSC) to detect exothermic/endothermic events .
Q. What strategies are effective for isotopic labeling of this compound to trace metabolic pathways?
- Methodological Answer : Incorporate deuterium (H) at the α-position via base-catalyzed H/D exchange using DO and NaOD. Confirm labeling efficiency (>98%) via mass spectrometry (MS) and H NMR. For C labeling, synthesize from C-enriched precursors (e.g., C-hexanoic acid) and validate using isotope ratio mass spectrometry (IRMS) .
Q. How can researchers design experiments to investigate this compound’s synergistic effects with other esters?
- Methodological Answer : Use a factorial design to test binary mixtures with esters like ethyl acetate or methyl octanoate. Measure synergistic parameters (e.g., vapor-liquid equilibrium shifts) via headspace GC. Control variables include molar ratios (1:1 to 1:5) and temperature (25–50°C). Statistical models like response surface methodology (RSM) quantify interaction effects .
Q. Data Analysis and Validation
Q. What statistical methods are critical for analyzing this compound’s solvent effects on reaction kinetics?
- Methodological Answer : Fit kinetic data (e.g., rate constants) to the Eyring-Polanyi equation using nonlinear regression. Compare solvent polarity (via Kamlet-Taft parameters) using multivariate analysis. Report confidence intervals (95%) and p-values to validate solvent-dependent trends. Outliers should be scrutinized for solvent purity issues (e.g., peroxide content in ethers) .
Q. How should researchers validate computational models predicting this compound’s spectroscopic properties?
- Methodological Answer : Compare density functional theory (DFT)-calculated IR/NMR spectra with experimental data. Use root-mean-square deviation (RMSD) analysis for vibrational modes (<10 cm acceptable) and chemical shifts (<0.5 ppm for H NMR). Validate solvation models (e.g., COSMO-RS) against experimental dielectric constant-dependent data .
Q. What protocols ensure reproducibility in this compound’s degradation studies under UV light?
- Methodological Answer : Use a calibrated UV chamber (λ = 254 nm, intensity 1.5 mW/cm) and monitor irradiance with a radiometer. Quantify degradation products (e.g., 4-oxononanoic acid) via LC-MS/MS. Include control samples shielded from UV to rule out thermal degradation. Report photon flux and quantum yield calculations to enable cross-lab comparisons .
Q. Experimental Design Pitfalls
Q. What are common errors in designing dose-response studies with this compound?
- Methodological Answer : Avoid oversaturating biological systems by testing a logarithmic concentration range (e.g., 1 nM–100 μM). Normalize responses to vehicle controls (e.g., DMSO <0.1%). Use Hill slope analysis to detect cooperative effects. Replicate experiments across cell lines or in vivo models to confirm mechanistic specificity .
Q. How can researchers mitigate batch-to-batch variability in this compound synthesis?
- Methodological Answer : Standardize precursor sourcing (e.g., CAS-certified hexanoic acid) and reaction conditions (e.g., strict temperature control ±1°C). Implement quality control (QC) checks via H NMR integration of key protons (e.g., ethyl ester CH) to ensure >98% consistency. Document deviations in lab notebooks for traceability .
Q. Data Presentation and Reproducibility
Q. How should researchers present conflicting spectral data for this compound in publications?
- Methodological Answer : Include raw spectra in supplementary materials with baseline correction annotations. Use overlays to highlight discrepancies and discuss potential causes (e.g., solvent impurities, instrumental drift). Reference analogous compounds’ spectra from peer-reviewed databases like SDBS .
Q. What open-science practices enhance the reproducibility of this compound research?
- Methodological Answer : Deposit raw NMR, MS, and chromatographic data in repositories like Zenodo or Figshare with CC-BY licenses. Provide detailed metadata (e.g., instrument calibration logs, solvent batch numbers). Adopt FAIR principles (Findable, Accessible, Interoperable, Reusable) for all datasets .
Properties
IUPAC Name |
ethyl 4-oxononanoate | |
---|---|---|
Source | PubChem | |
URL | https://pubchem.ncbi.nlm.nih.gov | |
Description | Data deposited in or computed by PubChem | |
InChI |
InChI=1S/C11H20O3/c1-3-5-6-7-10(12)8-9-11(13)14-4-2/h3-9H2,1-2H3 | |
Source | PubChem | |
URL | https://pubchem.ncbi.nlm.nih.gov | |
Description | Data deposited in or computed by PubChem | |
InChI Key |
RHHOUINGCOVIBN-UHFFFAOYSA-N | |
Source | PubChem | |
URL | https://pubchem.ncbi.nlm.nih.gov | |
Description | Data deposited in or computed by PubChem | |
Canonical SMILES |
CCCCCC(=O)CCC(=O)OCC | |
Source | PubChem | |
URL | https://pubchem.ncbi.nlm.nih.gov | |
Description | Data deposited in or computed by PubChem | |
Molecular Formula |
C11H20O3 | |
Source | PubChem | |
URL | https://pubchem.ncbi.nlm.nih.gov | |
Description | Data deposited in or computed by PubChem | |
DSSTOX Substance ID |
DTXSID80498380 | |
Record name | Ethyl 4-oxononanoate | |
Source | EPA DSSTox | |
URL | https://comptox.epa.gov/dashboard/DTXSID80498380 | |
Description | DSSTox provides a high quality public chemistry resource for supporting improved predictive toxicology. | |
Molecular Weight |
200.27 g/mol | |
Source | PubChem | |
URL | https://pubchem.ncbi.nlm.nih.gov | |
Description | Data deposited in or computed by PubChem | |
CAS No. |
37174-92-8 | |
Record name | Ethyl 4-oxononanoate | |
Source | EPA DSSTox | |
URL | https://comptox.epa.gov/dashboard/DTXSID80498380 | |
Description | DSSTox provides a high quality public chemistry resource for supporting improved predictive toxicology. | |
Retrosynthesis Analysis
AI-Powered Synthesis Planning: Our tool employs the Template_relevance Pistachio, Template_relevance Bkms_metabolic, Template_relevance Pistachio_ringbreaker, Template_relevance Reaxys, Template_relevance Reaxys_biocatalysis model, leveraging a vast database of chemical reactions to predict feasible synthetic routes.
One-Step Synthesis Focus: Specifically designed for one-step synthesis, it provides concise and direct routes for your target compounds, streamlining the synthesis process.
Accurate Predictions: Utilizing the extensive PISTACHIO, BKMS_METABOLIC, PISTACHIO_RINGBREAKER, REAXYS, REAXYS_BIOCATALYSIS database, our tool offers high-accuracy predictions, reflecting the latest in chemical research and data.
Strategy Settings
Precursor scoring | Relevance Heuristic |
---|---|
Min. plausibility | 0.01 |
Model | Template_relevance |
Template Set | Pistachio/Bkms_metabolic/Pistachio_ringbreaker/Reaxys/Reaxys_biocatalysis |
Top-N result to add to graph | 6 |
Feasible Synthetic Routes
Disclaimer and Information on In-Vitro Research Products
Please be aware that all articles and product information presented on BenchChem are intended solely for informational purposes. The products available for purchase on BenchChem are specifically designed for in-vitro studies, which are conducted outside of living organisms. In-vitro studies, derived from the Latin term "in glass," involve experiments performed in controlled laboratory settings using cells or tissues. It is important to note that these products are not categorized as medicines or drugs, and they have not received approval from the FDA for the prevention, treatment, or cure of any medical condition, ailment, or disease. We must emphasize that any form of bodily introduction of these products into humans or animals is strictly prohibited by law. It is essential to adhere to these guidelines to ensure compliance with legal and ethical standards in research and experimentation.