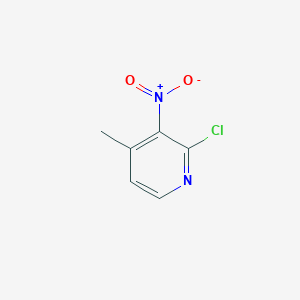
2-Chloro-4-methyl-3-nitropyridine
Overview
Description
2-Chloro-4-methyl-3-nitropyridine is a halogenated heterocyclic compound with the molecular formula C6H5ClN2O2 and a molecular weight of 172.57 g/mol . It is characterized by a pyridine ring substituted with a chlorine atom at the 2-position, a methyl group at the 4-position, and a nitro group at the 3-position . This compound is often used as an intermediate in the synthesis of pharmaceuticals and other organic compounds .
Mechanism of Action
Target of Action
2-Chloro-4-methyl-3-nitropyridine is a biochemical reagent . It is used as a biological material or organic compound for life science related research . It is a derivative of cyclopropyldipyridodiazepinone and is used as non-nucleoside reverse transcriptase inhibitors .
Mode of Action
The compound interacts directly with HIV-1’s reverse transcriptase . It works by breaking the catalytic end of this enzyme, thereby inhibiting both RNA-dependent and DNA-dependent DNA polymerase activity . This effectively reduces the number of viruses in the body and restores the body’s immune function .
Pharmacokinetics
The compound’s solubility in chloroform, dmso, and methanol suggests that it may have good bioavailability.
Result of Action
The primary result of the action of this compound is the reduction of the number of viruses in the body and the restoration of the body’s immune function . By inhibiting the reverse transcription process, it prevents the replication of HIV-1, thereby reducing the viral load.
Action Environment
For instance, the compound should be stored in a dark place, sealed, and in dry conditions .
Preparation Methods
Synthetic Routes and Reaction Conditions: The synthesis of 2-Chloro-4-methyl-3-nitropyridine typically involves the chlorination of 3-nitro-4-methylpyridine-2-one. The process includes the following steps :
Preparation of 3-nitro-4-methylpyridine-2-one: This intermediate is synthesized by reacting 2-nitroacetic acid ethyl ester with 2-bromocrotonaldehyde in the presence of a base catalyst such as DBU (1,8-Diazabicyclo[5.4.0]undec-7-ene) at 40-45°C for 5 hours.
Chlorination: The 3-nitro-4-methylpyridine-2-one is then chlorinated using phosphorus oxychloride (POCl3) at 70-75°C for 8 hours.
Industrial Production Methods: Industrial production methods for this compound are similar to the laboratory synthesis but are scaled up to accommodate larger quantities. The use of continuous flow reactors and automated systems can enhance the efficiency and yield of the production process .
Chemical Reactions Analysis
Types of Reactions: 2-Chloro-4-methyl-3-nitropyridine undergoes various chemical reactions, including:
Nucleophilic Substitution: The chlorine atom can be substituted by nucleophiles such as amines, thiols, and alkoxides.
Oxidation: The methyl group can be oxidized to a carboxylic acid using strong oxidizing agents.
Common Reagents and Conditions:
Nucleophilic Substitution: Reagents such as sodium methoxide, sodium thiolate, and primary amines are commonly used under basic conditions.
Oxidation: Potassium permanganate (KMnO4) or chromium trioxide (CrO3) are used for the oxidation of the methyl group.
Major Products:
Scientific Research Applications
2-Chloro-4-methyl-3-nitropyridine has several scientific research applications:
Comparison with Similar Compounds
- 2-Chloro-5-methyl-3-nitropyridine
- 2-Chloro-3-methyl-5-nitropyridine
- 2-Amino-4-methyl-3-nitropyridine
Comparison:
- 2-Chloro-5-methyl-3-nitropyridine: Similar structure but with the methyl group at the 5-position instead of the 4-position. This positional isomer may exhibit different reactivity and biological activity .
- 2-Chloro-3-methyl-5-nitropyridine: Another positional isomer with the nitro group at the 5-position. It may have different chemical properties and applications .
- 2-Amino-4-methyl-3-nitropyridine: Contains an amino group instead of a chlorine atom, which significantly alters its chemical reactivity and potential applications .
2-Chloro-4-methyl-3-nitropyridine is unique due to its specific substitution pattern, which imparts distinct chemical and biological properties .
Properties
IUPAC Name |
2-chloro-4-methyl-3-nitropyridine | |
---|---|---|
Source | PubChem | |
URL | https://pubchem.ncbi.nlm.nih.gov | |
Description | Data deposited in or computed by PubChem | |
InChI |
InChI=1S/C6H5ClN2O2/c1-4-2-3-8-6(7)5(4)9(10)11/h2-3H,1H3 | |
Source | PubChem | |
URL | https://pubchem.ncbi.nlm.nih.gov | |
Description | Data deposited in or computed by PubChem | |
InChI Key |
JHARVUVBTAAPLA-UHFFFAOYSA-N | |
Source | PubChem | |
URL | https://pubchem.ncbi.nlm.nih.gov | |
Description | Data deposited in or computed by PubChem | |
Canonical SMILES |
CC1=C(C(=NC=C1)Cl)[N+](=O)[O-] | |
Source | PubChem | |
URL | https://pubchem.ncbi.nlm.nih.gov | |
Description | Data deposited in or computed by PubChem | |
Molecular Formula |
C6H5ClN2O2 | |
Source | PubChem | |
URL | https://pubchem.ncbi.nlm.nih.gov | |
Description | Data deposited in or computed by PubChem | |
DSSTOX Substance ID |
DTXSID30323072 | |
Record name | 2-Chloro-4-methyl-3-nitropyridine | |
Source | EPA DSSTox | |
URL | https://comptox.epa.gov/dashboard/DTXSID30323072 | |
Description | DSSTox provides a high quality public chemistry resource for supporting improved predictive toxicology. | |
Molecular Weight |
172.57 g/mol | |
Source | PubChem | |
URL | https://pubchem.ncbi.nlm.nih.gov | |
Description | Data deposited in or computed by PubChem | |
CAS No. |
23056-39-5 | |
Record name | 2-Chloro-4-methyl-3-nitropyridine | |
Source | CAS Common Chemistry | |
URL | https://commonchemistry.cas.org/detail?cas_rn=23056-39-5 | |
Description | CAS Common Chemistry is an open community resource for accessing chemical information. Nearly 500,000 chemical substances from CAS REGISTRY cover areas of community interest, including common and frequently regulated chemicals, and those relevant to high school and undergraduate chemistry classes. This chemical information, curated by our expert scientists, is provided in alignment with our mission as a division of the American Chemical Society. | |
Explanation | The data from CAS Common Chemistry is provided under a CC-BY-NC 4.0 license, unless otherwise stated. | |
Record name | NSC 402977 | |
Source | ChemIDplus | |
URL | https://pubchem.ncbi.nlm.nih.gov/substance/?source=chemidplus&sourceid=0023056395 | |
Description | ChemIDplus is a free, web search system that provides access to the structure and nomenclature authority files used for the identification of chemical substances cited in National Library of Medicine (NLM) databases, including the TOXNET system. | |
Record name | 23056-39-5 | |
Source | DTP/NCI | |
URL | https://dtp.cancer.gov/dtpstandard/servlet/dwindex?searchtype=NSC&outputformat=html&searchlist=402977 | |
Description | The NCI Development Therapeutics Program (DTP) provides services and resources to the academic and private-sector research communities worldwide to facilitate the discovery and development of new cancer therapeutic agents. | |
Explanation | Unless otherwise indicated, all text within NCI products is free of copyright and may be reused without our permission. Credit the National Cancer Institute as the source. | |
Record name | 2-Chloro-4-methyl-3-nitropyridine | |
Source | EPA DSSTox | |
URL | https://comptox.epa.gov/dashboard/DTXSID30323072 | |
Description | DSSTox provides a high quality public chemistry resource for supporting improved predictive toxicology. | |
Synthesis routes and methods
Procedure details
Retrosynthesis Analysis
AI-Powered Synthesis Planning: Our tool employs the Template_relevance Pistachio, Template_relevance Bkms_metabolic, Template_relevance Pistachio_ringbreaker, Template_relevance Reaxys, Template_relevance Reaxys_biocatalysis model, leveraging a vast database of chemical reactions to predict feasible synthetic routes.
One-Step Synthesis Focus: Specifically designed for one-step synthesis, it provides concise and direct routes for your target compounds, streamlining the synthesis process.
Accurate Predictions: Utilizing the extensive PISTACHIO, BKMS_METABOLIC, PISTACHIO_RINGBREAKER, REAXYS, REAXYS_BIOCATALYSIS database, our tool offers high-accuracy predictions, reflecting the latest in chemical research and data.
Strategy Settings
Precursor scoring | Relevance Heuristic |
---|---|
Min. plausibility | 0.01 |
Model | Template_relevance |
Template Set | Pistachio/Bkms_metabolic/Pistachio_ringbreaker/Reaxys/Reaxys_biocatalysis |
Top-N result to add to graph | 6 |
Feasible Synthetic Routes
Q1: What are the key structural features of 2-chloro-4-methyl-3-nitropyridine, and how were they investigated in the study?
A1: The provided research paper focuses on a comparative study of this compound and 2-chloro-6-methylpyridine. [] The study primarily utilizes vibrational spectroscopy, conformational analysis, and electronic structure calculations to compare and contrast the two compounds. While it doesn't delve into specific applications of this compound, the study provides crucial information about its structure and behavior.
Disclaimer and Information on In-Vitro Research Products
Please be aware that all articles and product information presented on BenchChem are intended solely for informational purposes. The products available for purchase on BenchChem are specifically designed for in-vitro studies, which are conducted outside of living organisms. In-vitro studies, derived from the Latin term "in glass," involve experiments performed in controlled laboratory settings using cells or tissues. It is important to note that these products are not categorized as medicines or drugs, and they have not received approval from the FDA for the prevention, treatment, or cure of any medical condition, ailment, or disease. We must emphasize that any form of bodily introduction of these products into humans or animals is strictly prohibited by law. It is essential to adhere to these guidelines to ensure compliance with legal and ethical standards in research and experimentation.