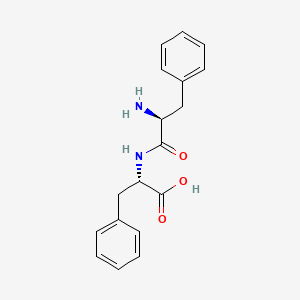
H-Phe-phe-OH
Overview
Description
H-Phe-Phe-OH, also known as phenylalanyl-phenylalanine, is a dipeptide composed of two phenylalanine molecules. Phenylalanine is an essential amino acid and a precursor for the amino acid tyrosine.
Scientific Research Applications
H-Phe-Phe-OH has a wide range of scientific research applications:
Chemistry: Used as a building block for synthesizing more complex peptides and proteins.
Biology: Studied for its role in protein-protein interactions and enzyme-substrate recognition.
Medicine: Investigated for its potential in drug delivery systems and as a therapeutic agent.
Industry: Utilized in the development of biomaterials, sensors, and bioelectronics
Mechanism of Action
Target of Action
H-Phe-Phe-OH, also known as Phenylalanyl-phenylalanine or Phe-Phe, is a short peptide composed of two phenylalanine molecules . Phenylalanine is an essential amino acid and the precursor for the amino acid tyrosine .
Mode of Action
The mode of action of this compound is primarily through its role in protein synthesis. As a dipeptide of phenylalanine, it can be incorporated into proteins during translation, influencing the structure and function of these proteins . Additionally, this compound has been found to self-assemble into nanostructures and hydrogels, which could have potential applications in nanomedicine .
Biochemical Pathways
Phenylalanine, the building block of this compound, is a precursor for the amino acid tyrosine . Tyrosine is involved in several biochemical pathways, including the synthesis of catecholamines (neurotransmitters such as dopamine, norepinephrine, and epinephrine) in the body . Therefore, this compound may indirectly influence these pathways through its role in protein synthesis.
Result of Action
The result of this compound’s action is primarily its incorporation into proteins, influencing their structure and function . Additionally, its ability to self-assemble into nanostructures and hydrogels could have potential applications in drug delivery and biomaterials .
Action Environment
The action of this compound can be influenced by various environmental factors. For instance, the pH and temperature can affect the rate of peptide bond formation and breakage, thereby influencing the stability of this compound. Furthermore, the presence of other molecules can impact the self-assembly of this compound into nanostructures and hydrogels .
Biochemical Analysis
Biochemical Properties
H-Phe-phe-OH plays a significant role in various biochemical reactions. It interacts with several enzymes, proteins, and other biomolecules. For instance, this compound can act as a substrate for enzymes involved in peptide bond formation and hydrolysis. It also interacts with proteins that recognize and bind to phenylalanine residues, influencing protein folding and stability. Additionally, this compound can form non-covalent interactions with other biomolecules, such as nucleic acids, through aromatic stacking and hydrogen bonding .
Cellular Effects
This compound affects various types of cells and cellular processes. It can influence cell function by modulating cell signaling pathways, gene expression, and cellular metabolism. For example, this compound can activate or inhibit specific signaling pathways by binding to receptors or enzymes involved in these pathways. This can lead to changes in gene expression, affecting the production of proteins and other molecules essential for cellular function. Moreover, this compound can impact cellular metabolism by altering the activity of metabolic enzymes and the availability of metabolic intermediates .
Molecular Mechanism
The molecular mechanism of this compound involves its interactions with biomolecules at the molecular level. This compound can bind to enzymes, either inhibiting or activating their activity. This binding can occur through various interactions, such as hydrogen bonding, hydrophobic interactions, and aromatic stacking. Additionally, this compound can influence gene expression by interacting with transcription factors or other regulatory proteins. These interactions can lead to changes in the transcriptional activity of specific genes, ultimately affecting cellular function .
Temporal Effects in Laboratory Settings
In laboratory settings, the effects of this compound can change over time. The stability and degradation of this compound are crucial factors that influence its long-term effects on cellular function. Studies have shown that this compound is relatively stable under physiological conditions, but it can degrade over time, leading to a decrease in its activity. Long-term exposure to this compound can result in sustained changes in cellular function, including alterations in gene expression and metabolic activity .
Dosage Effects in Animal Models
The effects of this compound vary with different dosages in animal models. At low doses, this compound may have minimal effects on cellular function, while higher doses can lead to significant changes in cell signaling pathways, gene expression, and metabolism. Threshold effects have been observed, where a certain dosage is required to elicit a measurable response. At very high doses, this compound can exhibit toxic or adverse effects, such as cellular stress, apoptosis, or necrosis .
Metabolic Pathways
This compound is involved in several metabolic pathways, interacting with various enzymes and cofactors. It can be metabolized by enzymes that cleave peptide bonds, releasing free phenylalanine molecules. These phenylalanine molecules can then enter metabolic pathways, such as the synthesis of tyrosine or the production of neurotransmitters like dopamine and norepinephrine. This compound can also affect metabolic flux and metabolite levels by modulating the activity of key metabolic enzymes .
Transport and Distribution
This compound is transported and distributed within cells and tissues through various mechanisms. It can be taken up by cells via specific transporters or endocytosis. Once inside the cell, this compound can interact with binding proteins that facilitate its transport to specific cellular compartments. The localization and accumulation of this compound within cells can influence its activity and function, affecting cellular processes such as signaling and metabolism .
Subcellular Localization
The subcellular localization of this compound is essential for its activity and function. This compound can be directed to specific compartments or organelles through targeting signals or post-translational modifications. For example, this compound may be localized to the cytoplasm, nucleus, or mitochondria, depending on its interactions with other biomolecules. The subcellular localization of this compound can influence its ability to modulate cellular processes, such as gene expression, signaling, and metabolism .
Preparation Methods
Synthetic Routes and Reaction Conditions
H-Phe-Phe-OH can be synthesized using solid-phase peptide synthesis (SPPS), a common method for producing peptides. The process involves the sequential addition of protected amino acids to a growing peptide chain anchored to a solid resin. The protecting groups are removed, and the peptide is cleaved from the resin to yield the final product .
Industrial Production Methods
Industrial production of this compound typically involves large-scale SPPS. This method allows for the efficient and high-yield production of peptides. The process can be optimized by adjusting reaction conditions such as temperature, solvent, and pH to enhance the purity and yield of the final product .
Chemical Reactions Analysis
Types of Reactions
H-Phe-Phe-OH undergoes various chemical reactions, including:
Oxidation: The phenylalanine residues can be oxidized to form quinones.
Reduction: Reduction reactions can convert quinones back to phenols.
Substitution: The aromatic rings of phenylalanine can undergo electrophilic aromatic substitution reactions.
Common Reagents and Conditions
Oxidation: Common oxidizing agents include potassium permanganate and hydrogen peroxide.
Reduction: Reducing agents such as sodium borohydride and lithium aluminum hydride are used.
Substitution: Electrophilic aromatic substitution reactions often use reagents like bromine or chlorine in the presence of a Lewis acid catalyst.
Major Products Formed
Oxidation: Quinones and other oxidized derivatives.
Reduction: Phenols and reduced derivatives.
Substitution: Halogenated phenylalanine derivatives.
Comparison with Similar Compounds
Similar Compounds
H-Tyr-Tic-Phe-Phe-OH: A selective δ-opioid receptor ligand with unique agonist and antagonist properties.
H-Phe-Phe-Phe-OH: An aromatic tripeptide used as a DNA molecular probe.
Boc-Phe-Phe-OH: A protected diphenylalanine scaffold used in the study of self-assembly and nanostructure formation.
Uniqueness
H-Phe-Phe-OH is unique due to its simplicity and versatility as a dipeptide. Its ability to self-assemble into various nanostructures makes it a valuable tool in nanomedicine and biomaterial research. The compound’s straightforward synthesis and modification potential further enhance its utility in scientific research .
Properties
IUPAC Name |
2-[(2-amino-3-phenylpropanoyl)amino]-3-phenylpropanoic acid | |
---|---|---|
Details | Computed by LexiChem 2.6.6 (PubChem release 2019.06.18) | |
Source | PubChem | |
URL | https://pubchem.ncbi.nlm.nih.gov | |
Description | Data deposited in or computed by PubChem | |
InChI |
InChI=1S/C18H20N2O3/c19-15(11-13-7-3-1-4-8-13)17(21)20-16(18(22)23)12-14-9-5-2-6-10-14/h1-10,15-16H,11-12,19H2,(H,20,21)(H,22,23) | |
Details | Computed by InChI 1.0.5 (PubChem release 2019.06.18) | |
Source | PubChem | |
URL | https://pubchem.ncbi.nlm.nih.gov | |
Description | Data deposited in or computed by PubChem | |
InChI Key |
GKZIWHRNKRBEOH-UHFFFAOYSA-N | |
Details | Computed by InChI 1.0.5 (PubChem release 2019.06.18) | |
Source | PubChem | |
URL | https://pubchem.ncbi.nlm.nih.gov | |
Description | Data deposited in or computed by PubChem | |
Canonical SMILES |
C1=CC=C(C=C1)CC(C(=O)NC(CC2=CC=CC=C2)C(=O)O)N | |
Details | Computed by OEChem 2.1.5 (PubChem release 2019.06.18) | |
Source | PubChem | |
URL | https://pubchem.ncbi.nlm.nih.gov | |
Description | Data deposited in or computed by PubChem | |
Molecular Formula |
C18H20N2O3 | |
Details | Computed by PubChem 2.1 (PubChem release 2019.06.18) | |
Source | PubChem | |
URL | https://pubchem.ncbi.nlm.nih.gov | |
Description | Data deposited in or computed by PubChem | |
Molecular Weight |
312.4 g/mol | |
Details | Computed by PubChem 2.1 (PubChem release 2021.05.07) | |
Source | PubChem | |
URL | https://pubchem.ncbi.nlm.nih.gov | |
Description | Data deposited in or computed by PubChem | |
CAS No. |
2577-40-4 | |
Record name | Phenylalanylphenylalanine | |
Source | ChemIDplus | |
URL | https://pubchem.ncbi.nlm.nih.gov/substance/?source=chemidplus&sourceid=0002577404 | |
Description | ChemIDplus is a free, web search system that provides access to the structure and nomenclature authority files used for the identification of chemical substances cited in National Library of Medicine (NLM) databases, including the TOXNET system. | |
Record name | 2577-40-4 | |
Source | DTP/NCI | |
URL | https://dtp.cancer.gov/dtpstandard/servlet/dwindex?searchtype=NSC&outputformat=html&searchlist=524136 | |
Description | The NCI Development Therapeutics Program (DTP) provides services and resources to the academic and private-sector research communities worldwide to facilitate the discovery and development of new cancer therapeutic agents. | |
Explanation | Unless otherwise indicated, all text within NCI products is free of copyright and may be reused without our permission. Credit the National Cancer Institute as the source. | |
Record name | 3-phenyl-N-(3-phenyl-L-alanyl)-L-alanine | |
Source | European Chemicals Agency (ECHA) | |
URL | https://echa.europa.eu/substance-information/-/substanceinfo/100.018.119 | |
Description | The European Chemicals Agency (ECHA) is an agency of the European Union which is the driving force among regulatory authorities in implementing the EU's groundbreaking chemicals legislation for the benefit of human health and the environment as well as for innovation and competitiveness. | |
Explanation | Use of the information, documents and data from the ECHA website is subject to the terms and conditions of this Legal Notice, and subject to other binding limitations provided for under applicable law, the information, documents and data made available on the ECHA website may be reproduced, distributed and/or used, totally or in part, for non-commercial purposes provided that ECHA is acknowledged as the source: "Source: European Chemicals Agency, http://echa.europa.eu/". Such acknowledgement must be included in each copy of the material. ECHA permits and encourages organisations and individuals to create links to the ECHA website under the following cumulative conditions: Links can only be made to webpages that provide a link to the Legal Notice page. | |
Retrosynthesis Analysis
AI-Powered Synthesis Planning: Our tool employs the Template_relevance Pistachio, Template_relevance Bkms_metabolic, Template_relevance Pistachio_ringbreaker, Template_relevance Reaxys, Template_relevance Reaxys_biocatalysis model, leveraging a vast database of chemical reactions to predict feasible synthetic routes.
One-Step Synthesis Focus: Specifically designed for one-step synthesis, it provides concise and direct routes for your target compounds, streamlining the synthesis process.
Accurate Predictions: Utilizing the extensive PISTACHIO, BKMS_METABOLIC, PISTACHIO_RINGBREAKER, REAXYS, REAXYS_BIOCATALYSIS database, our tool offers high-accuracy predictions, reflecting the latest in chemical research and data.
Strategy Settings
Precursor scoring | Relevance Heuristic |
---|---|
Min. plausibility | 0.01 |
Model | Template_relevance |
Template Set | Pistachio/Bkms_metabolic/Pistachio_ringbreaker/Reaxys/Reaxys_biocatalysis |
Top-N result to add to graph | 6 |
Feasible Synthetic Routes
Q1: How does H-Phe-Phe-OH contribute to the development of molecularly imprinted polymers (MIPs) for sensing applications?
A: this compound, or diphenylalanine, is a dipeptide that readily self-assembles into distinct nanostructures due to its inherent properties like hydrogen bonding and π-π stacking interactions. [, ] In the context of MIPs, this compound serves as a building block for creating the recognition sites within the polymer matrix. While not directly involved in binding the target molecule, this compound influences the polymer's morphology and potentially enhances the accessibility of the imprinted cavities. []
Q2: What are the potential advantages of incorporating this compound into sensing platforms for molecules like ethyl carbamate?
A2: Incorporating this compound into sensing platforms offers several potential advantages, particularly in conjunction with fluorescent molecules like genipin:
- Enhanced Sensitivity: The self-assembly of this compound can contribute to a well-defined nanostructure within the sensing platform. This organized structure might enhance the interaction between the fluorescent core and the target molecule, potentially leading to improved sensitivity. []
- Dual-Signal Response: The combination of this compound and genipin in the optosensing core enables a dual-band fluorescence response. This dual signal can be advantageous for improving the accuracy and reliability of detection by providing two independent measurement points. []
- Biocompatibility: As a peptide-based building block, this compound offers potential biocompatibility, which can be beneficial for applications involving biological samples or in vivo sensing. []
Disclaimer and Information on In-Vitro Research Products
Please be aware that all articles and product information presented on BenchChem are intended solely for informational purposes. The products available for purchase on BenchChem are specifically designed for in-vitro studies, which are conducted outside of living organisms. In-vitro studies, derived from the Latin term "in glass," involve experiments performed in controlled laboratory settings using cells or tissues. It is important to note that these products are not categorized as medicines or drugs, and they have not received approval from the FDA for the prevention, treatment, or cure of any medical condition, ailment, or disease. We must emphasize that any form of bodily introduction of these products into humans or animals is strictly prohibited by law. It is essential to adhere to these guidelines to ensure compliance with legal and ethical standards in research and experimentation.