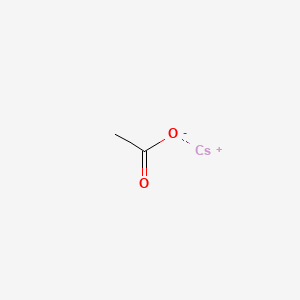
Cesium acetate
- Click on QUICK INQUIRY to receive a quote from our team of experts.
- With the quality product at a COMPETITIVE price, you can focus more on your research.
Overview
Description
Cesium acetate, also known as cesium ethanoate, is an ionic compound with the molecular formula CH₃COOCs. It is a white, hygroscopic solid that is highly soluble in water. This compound is primarily used in organic synthesis and has applications in various scientific fields due to its unique chemical properties .
Mechanism of Action
- Role : CsAc helps enhance the stability and efficiency of perovskite solar cells by modifying the crystal structure and reducing trap density in the resulting perovskite layers .
- The incorporation of CsAc extends carrier lifetime and reduces hysteresis phenomena in perovskite solar cells .
- CsAc modifies this process, allowing stable α-FAPbI₃ black phase formation by structurally engineering the metal halide layer and precise perovskite composition .
Target of Action
Mode of Action
Biochemical Pathways
Result of Action
Biochemical Analysis
Biochemical Properties
Cesium acetate plays a role in biochemical reactions, particularly in organic synthesis. It is known to interact with enzymes and proteins involved in glycosylation reactions. For example, this compound has been shown to promote the glycosylation of nucleosides, which is crucial in nucleic acid research and drug development . The interaction between this compound and enzymes in these reactions enhances the efficiency and yield of the desired products.
Cellular Effects
This compound influences various cellular processes. It has been observed to affect cell signaling pathways, gene expression, and cellular metabolism. In particular, this compound can alter the pH of cellular environments, which in turn affects enzyme activity and metabolic processes . This compound’s impact on cellular function is significant, as it can modulate the activity of key enzymes and proteins involved in critical cellular pathways.
Molecular Mechanism
The molecular mechanism of this compound involves its interaction with biomolecules at the molecular level. This compound can bind to enzymes and proteins, leading to either inhibition or activation of their functions. For instance, in glycosylation reactions, this compound acts as a catalyst, promoting the formation of glycosidic bonds . Additionally, this compound can influence gene expression by altering the cellular pH, which affects the activity of transcription factors and other regulatory proteins.
Temporal Effects in Laboratory Settings
In laboratory settings, the effects of this compound can change over time. The stability and degradation of this compound are important factors to consider. This compound is relatively stable under standard laboratory conditions, but its long-term effects on cellular function can vary. Studies have shown that prolonged exposure to this compound can lead to changes in cellular metabolism and enzyme activity . These temporal effects are crucial for understanding the compound’s long-term impact on biological systems.
Dosage Effects in Animal Models
The effects of this compound vary with different dosages in animal models. At low doses, this compound can enhance certain biochemical reactions without causing significant adverse effects. At high doses, this compound can exhibit toxic effects, including gastrointestinal distress, hypotension, and syncope . It is essential to determine the optimal dosage to maximize the beneficial effects while minimizing toxicity.
Metabolic Pathways
This compound is involved in various metabolic pathways. It interacts with enzymes and cofactors that play a role in metabolic flux and metabolite levels. For example, this compound can influence the activity of enzymes involved in the tricarboxylic acid cycle, affecting the production of key metabolites . Understanding these metabolic pathways is crucial for elucidating the compound’s role in cellular metabolism.
Transport and Distribution
This compound is transported and distributed within cells and tissues through specific transporters and binding proteins. These interactions affect the localization and accumulation of this compound in different cellular compartments. The transport and distribution of this compound are essential for its biological activity and its impact on cellular function .
Subcellular Localization
The subcellular localization of this compound is influenced by targeting signals and post-translational modifications. This compound can be directed to specific compartments or organelles, where it exerts its effects on enzyme activity and cellular processes. Understanding the subcellular localization of this compound is important for elucidating its mechanism of action and its role in cellular function .
Preparation Methods
Synthetic Routes and Reaction Conditions: Cesium acetate can be synthesized by reacting cesium hydroxide (CsOH) or cesium carbonate (Cs₂CO₃) with acetic acid (CH₃COOH). The reaction typically proceeds as follows: [ \text{CsOH} + \text{CH}_3\text{COOH} \rightarrow \text{CH}_3\text{COOCs} + \text{H}_2\text{O} ] [ \text{Cs}_2\text{CO}_3 + 2\text{CH}_3\text{COOH} \rightarrow 2\text{CH}_3\text{COOCs} + \text{H}_2\text{O} + \text{CO}_2 ]
Industrial Production Methods: In industrial settings, this compound is often produced by neutralizing cesium hydroxide with acetic acid. The reaction is carried out in aqueous solution, and the product is then crystallized and dried .
Types of Reactions:
Substitution Reactions: this compound is commonly used in nucleophilic substitution reactions.
Condensation Reactions: In the Perkin synthesis, this compound is used to form unsaturated cinnamic-type acids by condensing aromatic aldehydes with fatty acids.
Common Reagents and Conditions:
Reagents: Cesium hydroxide, acetic acid, mesylates, aromatic aldehydes, fatty acids.
Conditions: Reactions are typically carried out in aqueous solutions or organic solvents under controlled temperatures.
Major Products:
O-Acetates: Formed from the substitution reactions involving secondary alcohols.
Cinnamic-Type Acids: Produced in the Perkin synthesis using this compound.
Scientific Research Applications
Cesium acetate has a wide range of applications in scientific research:
Organic Synthesis: It is used as a reagent in various organic reactions, including the synthesis of beta-pyranosyladenine nucleosides and diketodioxinone reactivity tuning.
Photovoltaics: It is used as a dopant in perovskite solar cells to improve stability and efficiency.
Electrochemistry: this compound is investigated for its role in eco-friendly electrolytes for lithium-ion batteries.
Comparison with Similar Compounds
Cesium acetate can be compared with other acetate salts such as:
- Lithium Acetate (CH₃COOLi)
- Sodium Acetate (CH₃COONa)
- Potassium Acetate (CH₃COOK)
- Rubidium Acetate (CH₃COORb)
Uniqueness:
- Higher Solubility: this compound has higher solubility in water compared to other acetate salts.
- Enhanced Yields: It provides significantly higher yields in certain organic reactions, such as the Perkin synthesis, compared to sodium acetate .
- Catalytic Efficiency: this compound enhances catalytic properties more effectively than other acetate salts in specific reactions .
By understanding the unique properties and applications of this compound, researchers can leverage its potential in various scientific and industrial fields.
Properties
CAS No. |
3396-11-0 |
---|---|
Molecular Formula |
C2H4CsO2 |
Molecular Weight |
192.96 g/mol |
IUPAC Name |
cesium;acetate |
InChI |
InChI=1S/C2H4O2.Cs/c1-2(3)4;/h1H3,(H,3,4); |
InChI Key |
ISOFMMPYICGGSC-UHFFFAOYSA-N |
SMILES |
CC(=O)[O-].[Cs+] |
Canonical SMILES |
CC(=O)O.[Cs] |
Key on ui other cas no. |
3396-11-0 |
Origin of Product |
United States |
Retrosynthesis Analysis
AI-Powered Synthesis Planning: Our tool employs the Template_relevance Pistachio, Template_relevance Bkms_metabolic, Template_relevance Pistachio_ringbreaker, Template_relevance Reaxys, Template_relevance Reaxys_biocatalysis model, leveraging a vast database of chemical reactions to predict feasible synthetic routes.
One-Step Synthesis Focus: Specifically designed for one-step synthesis, it provides concise and direct routes for your target compounds, streamlining the synthesis process.
Accurate Predictions: Utilizing the extensive PISTACHIO, BKMS_METABOLIC, PISTACHIO_RINGBREAKER, REAXYS, REAXYS_BIOCATALYSIS database, our tool offers high-accuracy predictions, reflecting the latest in chemical research and data.
Strategy Settings
Precursor scoring | Relevance Heuristic |
---|---|
Min. plausibility | 0.01 |
Model | Template_relevance |
Template Set | Pistachio/Bkms_metabolic/Pistachio_ringbreaker/Reaxys/Reaxys_biocatalysis |
Top-N result to add to graph | 6 |
Feasible Synthetic Routes
Q1: How does cesium acetate enhance the performance of perovskite solar cells (PSCs)?
A1: this compound plays a multifaceted role in improving PSC performance.
Q2: How does this compound interact with kaolinite surfaces?
A2: this compound intercalates into the kaolinite structure, causing the layers to expand. This process involves the incorporation of water molecules. The acetate ions hydrogen bond with the inner surface hydroxyls of the kaolinite, leading to the appearance of new infrared hydroxyl stretching and deformation modes [].
Q3: What is the molecular formula and weight of this compound?
A3: The molecular formula of this compound is CsCH3COO, and its molecular weight is 191.95 g/mol.
Q4: What spectroscopic techniques are useful for characterizing this compound and its interactions?
A4: Several spectroscopic techniques provide valuable information:
- Infrared (IR) Spectroscopy: Reveals changes in hydroxyl stretching and deformation modes, indicating interactions between acetate ions and target molecules like kaolinite [, , ].
- Raman Spectroscopy: Provides complementary information to IR, particularly useful for studying the hydration sphere of the cesium cation and its effect on the hydroxyl groups of the target molecule [, ].
- X-ray Diffraction (XRD): Confirms the intercalation of this compound into layered structures like kaolinite and provides information about the interlayer spacing [, ].
- Nuclear Magnetic Resonance (NMR) Spectroscopy: In specific applications, like studying reaction mechanisms involving this compound, NMR can be employed to monitor the formation and disappearance of intermediates and products [].
Q5: How does the thermal stability of this compound compare to other alkali metal acetates?
A5: this compound exhibits distinct thermal decomposition behavior compared to potassium and rubidium acetates. This difference is evident in the variations observed in their thermographic and thermogravimetric analysis, as well as the products formed during their decomposition through ketonic and methane pathways [].
Q6: How does this compound contribute to organic reactions as a catalyst or reagent?
A6: this compound demonstrates versatility in organic synthesis:
Q7: What is the role of this compound in the synthesis of isoquinolines?
A7: this compound acts as a base in the rhodium(III)-catalyzed dehydrative C-C and C-N coupling of oximines and alkynes to produce isoquinolines. This reaction involves ortho C-H activation of oximines followed by functionalization with alkynes. The mild, redox-neutral conditions and tolerance to air and moisture make this synthetic route particularly appealing [].
Q8: How is this compound used in the study of zeolite catalysts?
A8: this compound serves as a precursor for generating basic sites in zeolite catalysts:
Q9: How does the presence of this compound affect the stability of perovskite materials?
A9: The incorporation of this compound enhances the stability of perovskite materials, particularly their resistance to thermal and light-induced degradation. This improved stability is attributed to several factors, including the relaxation of strain within the perovskite layer due to cesium incorporation and the formation of a stable intermediate phase between acetate ions and lead iodide [].
Disclaimer and Information on In-Vitro Research Products
Please be aware that all articles and product information presented on BenchChem are intended solely for informational purposes. The products available for purchase on BenchChem are specifically designed for in-vitro studies, which are conducted outside of living organisms. In-vitro studies, derived from the Latin term "in glass," involve experiments performed in controlled laboratory settings using cells or tissues. It is important to note that these products are not categorized as medicines or drugs, and they have not received approval from the FDA for the prevention, treatment, or cure of any medical condition, ailment, or disease. We must emphasize that any form of bodily introduction of these products into humans or animals is strictly prohibited by law. It is essential to adhere to these guidelines to ensure compliance with legal and ethical standards in research and experimentation.